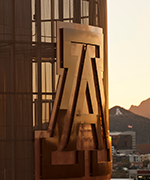
Anita A Koshy
- Professor
- Professor, Immunobiology
- Professor, BIO5 Institute
- Professor, Evelyn F McKnight Brain Institute
- Professor, Neuroscience - GIDP
- Member of the Graduate Faculty
- Professor, Medicine
Contact
- (520) 626-1696
- TW Keating Bioresearch Bldg., Rm. 0241
- Tucson, AZ 85721
- akoshy@arizona.edu
Degrees
- M.D. Medical Doctor
- Duke University, Durham, North Carolina, United States
- B.S. Biological Sciences
- Stanford University, Stanford, California, United States
Work Experience
- University of Arizona; Bio5 Institute Member, GIDP (2012 - Ongoing)
- Evelyn F. McKnight Brain Institute (2012 - Ongoing)
- University of Arizona; Department of Immunobiology (2012 - Ongoing)
- University of Arizona; Department of Neurology (2012 - Ongoing)
- Stanford University, Stanford, California (2010 - 2012)
- Infectious Disease, Stanford University (2006 - 2010)
Awards
- Engel Society
- Duke University Medical School, Fall 1997
- Howard Hughes Medical Institute Medical Student Fellowship
- Duke University Medical School, Fall 1997
- Outstanding Faculty Educator Award
- Department of Neurology, University of Arizona, Spring 2013
- Chief Fellow
- Division of Infectious Disease, Stanford University, Spring 2010
- AOA membership
- UCSF AOA chapter, Spring 2003
Licensure & Certification
- Diplomate, Neurology Boards, American Board of Psychiatry and Neurology, Diplomate, Neurology Boards, American Board of Psychiatry and Neurology (2006)
- Arizona Medical License, Arizona Medical License (2012)
- California Medical License (Retired status currently), California State Medical Board (2001)
Interests
No activities entered.
Courses
2024-25 Courses
-
Honors Directed Research
NROS 392H (Spring 2025) -
Honors Independent Study
PSIO 499H (Spring 2025) -
Honors Thesis
BIOC 498H (Spring 2025) -
Prins+Molec Mechanisms
IMB 565 (Spring 2025) -
Honors Independent Study
PCOL 499H (Fall 2024) -
Honors Thesis
BIOC 498H (Fall 2024) -
Neuro+Rehab Med Clerkshp
NEUR 813C (Fall 2024)
2023-24 Courses
-
Honors Independent Study
PCOL 399H (Spring 2024) -
Prins+Molec Mechanisms
IMB 565 (Spring 2024) -
Research
IMB 900 (Spring 2024) -
Senior Capstone
BIOC 498 (Spring 2024) -
Honors Directed Research
BIOC 392H (Fall 2023) -
Neuro+Rehab Med Clerkshp
NEUR 813C (Fall 2023) -
Research
IMB 900 (Fall 2023) -
Research
NRSC 900 (Fall 2023) -
Senior Capstone
BIOC 498 (Fall 2023)
2022-23 Courses
-
Dissertation
IMB 920 (Spring 2023) -
Honors Thesis
NSCS 498H (Spring 2023) -
Prins+Molec Mechanisms
IMB 565 (Spring 2023) -
Research
NRSC 900 (Spring 2023) -
Directed Research
MCB 792 (Fall 2022) -
Dissertation
IMB 920 (Fall 2022) -
Dissertation
NRSC 920 (Fall 2022) -
Honors Thesis
NSCS 498H (Fall 2022) -
Methods In Neuroscience
NRSC 700 (Fall 2022) -
Neuro+Rehab Med Clerkshp
NEUR 813C (Fall 2022) -
Research
NRSC 900 (Fall 2022)
2021-22 Courses
-
Dissertation
IMB 920 (Spring 2022) -
Dissertation
NRSC 920 (Spring 2022) -
Prins+Molec Mechanisms
IMB 565 (Spring 2022) -
Dissertation
IMB 920 (Fall 2021) -
Dissertation
NRSC 920 (Fall 2021) -
Honors Independent Study
NSCS 499H (Fall 2021) -
Methods In Neuroscience
NRSC 700 (Fall 2021) -
Neuro+Rehab Med Clerkshp
NEUR 813C (Fall 2021)
2020-21 Courses
-
Directed Research
MCB 792 (Spring 2021) -
Dissertation
IMB 920 (Spring 2021) -
Dissertation
NRSC 920 (Spring 2021) -
Neuro+Rehab Med Clerkshp
NEUR 813C (Spring 2021) -
Neurology Clerkship Clinical
NEUR 813C2 (Spring 2021) -
Prins+Molec Mechanisms
IMB 565 (Spring 2021) -
Directed Research
MCB 792 (Fall 2020) -
Dissertation
IMB 920 (Fall 2020) -
Dissertation
NRSC 920 (Fall 2020) -
Neuro+Rehab Med Clerkshp
NEUR 813C (Fall 2020)
2019-20 Courses
-
Dissertation
IMB 920 (Spring 2020) -
Dissertation
NRSC 920 (Spring 2020) -
Honors Directed Research
NSCS 492H (Spring 2020) -
Honors Independent Study
MCB 399H (Spring 2020) -
Honors Thesis
NSCS 498H (Spring 2020) -
Methods In Neuroscience
NRSC 700 (Spring 2020) -
Prins+Molec Mechanisms
IMB 565 (Spring 2020) -
Dissertation
IMB 920 (Fall 2019) -
Dissertation
NRSC 920 (Fall 2019) -
Honors Directed Research
NSCS 492H (Fall 2019) -
Honors Independent Study
MIC 399H (Fall 2019) -
Honors Independent Study
NSCS 199H (Fall 2019) -
Honors Thesis
NSCS 498H (Fall 2019) -
Internship in Applied Biosci
ABS 593A (Fall 2019) -
Neuro+Rehab Med Clerkshp
NEUR 813C (Fall 2019) -
Research
IMB 900 (Fall 2019)
2018-19 Courses
-
Directed Research
NSCS 392 (Spring 2019) -
Dissertation
IMB 920 (Spring 2019) -
Dissertation
NRSC 920 (Spring 2019) -
Honors Independent Study
NSCS 499H (Spring 2019) -
Internship in Applied Biosci
ABS 593A (Spring 2019) -
Methods In Neuroscience
NRSC 700 (Spring 2019) -
Prins+Molec Mechanisms
IMB 565 (Spring 2019) -
Research
NRSC 900 (Spring 2019) -
Dissertation
IMB 920 (Fall 2018) -
Dissertation
NRSC 920 (Fall 2018) -
Honors Independent Study
MCB 499H (Fall 2018) -
Methods In Neuroscience
NRSC 700 (Fall 2018) -
Neuro+Rehab Med Clerkshp
NEUR 813C (Fall 2018) -
Research
IMB 900 (Fall 2018)
2017-18 Courses
-
Directed Rsrch
MCB 492 (Spring 2018) -
Dissertation
IMB 920 (Spring 2018) -
Dissertation
NRSC 920 (Spring 2018) -
Honors Independent Study
NSCS 399H (Spring 2018) -
Honors Independent Study
NSCS 499H (Spring 2018) -
Honors Thesis
NSCS 498H (Spring 2018) -
Prins+Molec Mechanisms
IMB 565 (Spring 2018) -
Research
IMB 900 (Spring 2018) -
Directed Rsrch
MCB 492 (Fall 2017) -
Dissertation
IMB 920 (Fall 2017) -
Dissertation
NRSC 920 (Fall 2017) -
Honors Independent Study
MCB 499H (Fall 2017) -
Honors Independent Study
NSCS 499H (Fall 2017) -
Honors Thesis
NSCS 498H (Fall 2017) -
Introduction to Research
MCB 795A (Fall 2017) -
Neuro+Rehab Med Clerkshp
NEUR 813C (Fall 2017) -
Research
IMB 900 (Fall 2017)
2016-17 Courses
-
Dissertation
IMB 920 (Spring 2017) -
Dissertation
NRSC 920 (Spring 2017) -
Lab Presentations & Discussion
MCB 696A (Spring 2017) -
Neuro+Rehab Med Clerkshp
NEUR 813C (Spring 2017) -
Neurology Gen. Inpatient Svc.
NEUR 850A (Spring 2017) -
Prins+Molec Mechanisms
IMB 565 (Spring 2017) -
Research
IMB 900 (Spring 2017) -
Research
MCB 900 (Spring 2017) -
Dissertation
IMB 920 (Fall 2016) -
Lab Presentations & Discussion
MCB 696A (Fall 2016) -
Neuro+Rehab Med Clerkshp
NEUR 813C (Fall 2016) -
Research
IMB 900 (Fall 2016) -
Research
MCB 900 (Fall 2016) -
Scientific Grantsmanship
IMB 521 (Fall 2016)
2015-16 Courses
-
Dissertation
IMB 920 (Spring 2016) -
Honors Thesis
NSCS 498H (Spring 2016) -
Independent Study
BME 299 (Spring 2016) -
Independent Study
MIC 399 (Spring 2016) -
Introduction to Research
MCB 795A (Spring 2016) -
Neuro+Rehab Med Clerkshp
NEUR 813C (Spring 2016) -
Research
IMB 900 (Spring 2016) -
Research
NRSC 900 (Spring 2016)
Scholarly Contributions
Chapters
- Anderson, N. C., Koshy, A. A., & Roos, K. (2022). Infections of the Nervous System: Bacterial, Fungal, and Parasitic Diseases of the Nervous System. In Bradley’s Neurology in Clinical Practice. Elsevier.More infoThis is a chapter that I originally co-wrote in ~2010 (6th Edition) and then I help re-write/update every 4 years or so.
- Koshy, A. A. (2021). Parasitic Infections of the Central Nervous System. In Aminoff's Neurology and General Medicine, 6th Edition. Elsevier.
- Koshy, A. A., Harris, T. H., & Lodoen, M. B. (2020). Cerebral Toxoplasmosis. In Toxoplasma gondii: the model apicomplexan- Perspectives and Methods, 3rd Ed.
- Cabral, C. M., Merritt, E. F., & Koshy, A. A. (2019). Three-Dimensional Reconstruction of Toxoplasma–Neuron Interactions In Situ. In Methods in Molecular Biology(pp 283-295). doi:10.1007/978-1-4939-9857-9_16More infoHow tissue and cellular architecture affects host cell–microbe interactions in vivo remains poorly defined because imaging these interactions in complex tissue is difficult and standard in vitro cultures do not mimic whole organ architecture. Here we describe a method that combines new tissue clearing techniques, high-resolution imaging, and three-dimensional reconstruction to overcome these barriers and allow in situ imaging of host cell–microbe interactions in complex tissue. We use the interactions between neurons and Toxoplasma gondii, a ubiquitous, protozoan parasite that establish a lifelong central nervous system (CNS) infection in mice and humans, as a model for this technique. This method aims to provide an easy, reproducible way to visualize the complex relationship between host cells and intracellular pathogens within a whole organ.
- Koshy, A. A., Blackburn, B. G., & Singh, U. (2019). Free-Living Amebae. In Mandell, Douglas, and Bennett’s Principles and Practice of Infectious Diseases. Elsevier.
- Bonsell, K. C., & Koshy, A. A. (2018). Fever and Headache. In Emergency Management of Infectious Diseases, 2nd Ed(pp 50-63). Cambridge University Press.
- Bonsell, K. C., & Koshy, A. A. (2018). Meningitis. In Emergency Management of Infectious Diseases(pp 50-63). Cambridge University Press. doi:10.1017/9781316597095.009
Journals/Publications
- Bracha, S., Johnson, H. J., Pranckevicius, N. A., Catto, F., Economides, A. E., Litvinov, S., Hassi, K., Rigoli, M. T., Cheroni, C., Bonfanti, M., Valenti, A., Stucchi, S., Attreya, S., Ross, P. D., Walsh, D., Malachi, N., Livne, H., Eshel, R., Krupalnik, V., , Levin, D., et al. (2024).
Engineering Toxoplasma gondii secretion systems for intracellular delivery of multiple large therapeutic proteins to neurons
. Nature microbiology, 9(8), 2051-2072.More infoDelivering macromolecules across biological barriers such as the blood-brain barrier limits their application in vivo. Previous work has demonstrated that Toxoplasma gondii, a parasite that naturally travels from the human gut to the central nervous system (CNS), can deliver proteins to host cells. Here we engineered T. gondii's endogenous secretion systems, the rhoptries and dense granules, to deliver multiple large (>100 kDa) therapeutic proteins into neurons via translational fusions to toxofilin and GRA16. We demonstrate delivery in cultured cells, brain organoids and in vivo, and probe protein activity using imaging, pull-down assays, scRNA-seq and fluorescent reporters. We demonstrate robust delivery after intraperitoneal administration in mice and characterize 3D distribution throughout the brain. As proof of concept, we demonstrate GRA16-mediated brain delivery of the MeCP2 protein, a putative therapeutic target for Rett syndrome. By characterizing the potential and current limitations of the system, we aim to guide future improvements that will be required for broader application. - Merritt, E. F., Kochanowsky, J. A., Hervé, P., Watson, A. A., & Koshy, A. A. (2024). Toxoplasma type II effector GRA15 has limited influence in vivo. PLoS ONE, 19(3). doi:10.1371/journal.pone.0300764More infoToxoplasma gondii is an intracellular parasite that establishes a long-term infection in the brain of many warm-blooded hosts, including humans and rodents. Like all obligate intracellular microbes, Toxoplasma uses many effector proteins to manipulate the host cell to ensure parasite survival. While some of these effector proteins are universal to all Toxoplasma strains, some are polymorphic between Toxoplasma strains. One such polymorphic effector is GRA15. The gra15 allele carried by type II strains activates host NF-κB signaling, leading to the release of cytokines such as IL-12, TNF, and IL-1β from immune cells infected with type II parasites. Prior work also suggested that GRA15 promotes early host control of parasites in vivo, but the effect of GRA15 on parasite persistence in the brain and the peripheral immune response has not been well defined. For this reason, we sought to address this gap by generating a new IIΔgra15 strain and comparing outcomes at 3 weeks post infection between WT and IIΔgra15 infected mice. We found that the brain parasite burden and the number of macrophages/microglia and T cells in the brain did not differ between WT and IIΔgra15 infected mice. In addition, while IIΔgra15 infected mice had a lower number and frequency of splenic M1-like macrophages and frequency of PD-1+ CTLA-4+ CD4+ T cells and NK cells compared to WT infected mice, the IFN-γ+ CD4 and CD8 T cell populations were equivalent. In summary, our results suggest that in vivo GRA15 may have a subtle effect on the peripheral immune response, but this effect is not strong enough to alter brain parasite burden or parenchymal immune cell number at 3 weeks post infection.
- Shallberg, L. A., Eberhard, J. N., Winn, A., Chandrasekaran, S., Giuliano, C. J., Merritt, E. F., Willis, E., Christian, D. A., Aldridge, D. L., Bunkofske, M., Jacquet, M., Dzierszinski, F., Katifori, E., Lourido, S., Koshy, A. A., & Hunter, C. A. (2024). The latent stage of Toxoplasma gondii is targeted by the immune response and host protective. Biorxiv. doi:10.1101/2024.03.05.583527More infopre-print
- Kochanowsky, J. A., Chandrasekaran, S., Sanchez, J. R., Thomas, K. K., & Koshy, A. A. (2023). ROP16-mediated activation of STAT6 enhances cyst development of type III Toxoplasma gondii in neurons. PLoS pathogens, 19(4), e1011347.More infoToxoplasma gondii establishes a long-lived latent infection in the central nervous system (CNS) of its hosts. Reactivation in immunocompromised individuals can lead to life threatening disease. Latent infection is driven by the ability of the parasite to convert from the acute-stage tachyzoite to the latent-stage bradyzoite which resides in long-lived intracellular cysts. While much work has focused on the parasitic factors that drive cyst development, the host factors that influence encystment are not well defined. Here we show that a polymorphic secreted parasite kinase (ROP16), that phosphorylates host cell proteins, mediates efficient encystment of T. gondii in a stress-induced model of encystment and primary neuronal cell cultures (PNCs) in a strain-specific manner. Using short-hairpin RNA (shRNA) knockdowns in human foreskin fibroblasts (HFFs) and PNCs from transgenic mice, we determined that ROP16's cyst enhancing abilities are mediated, in part, by phosphorylation-and therefore activation-of the host cell transcription factor STAT6. To test the role of STAT6 in vivo, we infected wild-type (WT) and STAT6KO mice, finding that, compared to WT mice, STAT6KO mice have a decrease in CNS cyst burden but not overall parasite burden or dissemination to the CNS. Finally, we found a similar ROP16-dependent encystment defect in human pluripotent stem cell-derived neurons. Together, these findings identify a host cell factor (STAT6) that T. gondii manipulates in a strain-specific manner to generate a favorable encystment environment.
- Chandrasekaran, S., Kochanowsky, J. A., Merritt, E. F., Lagas, J. S., Swannigan, A., & Koshy, A. A. (2022). IFN-g stimulated murine and human neurons mount anti-parasitic defenses against the intracellular parasite Toxoplasma gondii. Nature Communication.More infoPosted to BioRxiv
- Kochanowsky, J. A., Thomas, K. K., & Koshy, A. A. (2021). ROP16-Mediated Activation of STAT6 Suppresses Host Cell Reactive Oxygen Species Production, Facilitating Type III Toxoplasma gondii Growth and Survival. mBio, 12(2).More infoPolymorphic effector proteins determine the susceptibility of strains to IFN-γ-mediated clearance mechanisms deployed by murine host cells. However, less is known about the influence of these polymorphic effector proteins on IFN-γ-independent clearance mechanisms. Here, we show that deletion of one such polymorphic effector protein, ROP16, from a type III background leads to a defect in parasite growth and survival in unstimulated human fibroblasts and murine macrophages. Rescue of these defects requires a ROP16 with a functional kinase domain and the ability to activate a specific family of host cell transcription factors (STAT3, 5a, and 6). The growth and survival defects correlate with an accumulation of host cell reactive oxygen species (ROS) and are prevented by treatment with an ROS inhibitor. Exogenous activation of STAT3 and 6 suppresses host cell ROS production during infection with ROP16-deficient parasites and depletion of STAT6, but not STAT3 or 5a, causes an accumulation of ROS in cells infected with wild-type parasites. Pharmacological inhibition of NOX2 and mitochondrially derived ROS also rescues growth and survival of ROP16-deficient parasites. Collectively, these findings reveal an IFN-γ-independent mechanism of parasite restriction in human cells that is subverted by injection of ROP16 by type III parasites. is an obligate intracellular parasite that infects up to one-third of the world's population. Control of the parasite is largely accomplished by IFN-γ-dependent mechanisms that stimulate innate and adaptive immune responses. Parasite suppression of IFN-γ-stimulated responses has been linked to proteins that the parasite secretes into its host cell. These secreted proteins vary by strain and determine strain-specific lethality in mice. How these strain-specific polymorphic effector proteins affect IFN-γ-independent parasite control mechanisms in human and murine cells is not well known. This study shows that one such secreted protein, ROP16, enables efficient parasite growth and survival by suppressing IFN-γ-independent production of ROS by human and mouse cells.
- Mendez, O. A., Flores Machado, E., Lu, J., & Koshy, A. A. (2021). Injection with protein affects neuron health and survival. eLife, 10.More infois an intracellular parasite that causes a long-term latent infection of neurons. Using a custom MATLAB-based mapping program in combination with a mouse model that allows us to permanently mark neurons injected with parasite proteins, we found that -injected neurons (TINs) are heterogeneously distributed in the brain, primarily localizing to the cortex followed by the striatum. In addition, we determined that cortical TINs are commonly (>50%) excitatory neurons (FoxP2) and that striatal TINs are often (>65%) medium spiny neurons (MSNs) (FoxP2). By performing single neuron patch clamping on striatal TINs and neighboring uninfected MSNs, we discovered that TINs have highly aberrant electrophysiology. As approximately 90% of TINs will die by 8 weeks post-infection, this abnormal physiology suggests that injection with protein-either directly or indirectly-affects neuronal health and survival. Collectively, these data offer the first insights into which neurons interact with and how these interactions alter neuron physiology in vivo.
- Chen, L., Christian, D. A., Kochanowsky, J. A., Phan, A. T., Clark, J. T., Wang, S., Berry, C., Oh, J., Chen, X., Roos, D. S., Beiting, D. P., Koshy, A. A., & Hunter, C. A. (2020). The Toxoplasma gondii virulence factor ROP16 acts in cis and trans, and suppresses T cell responses. The Journal of experimental medicine, 217(3).More infoThe ability of Toxoplasma gondii to inject the rhoptry kinase ROP16 into host cells results in the activation of the transcription factors STAT3 and STAT6, but it is unclear how these events impact infection. Here, parasites that inject Cre-recombinase with rhoptry proteins were used to distinguish infected macrophages from those only injected with parasite proteins. Transcriptional profiling revealed that injection of rhoptry proteins alone was sufficient to induce an M2 phenotype that is dependent on STAT3 and STAT6, but only infected cells displayed reduced expression of genes associated with antimicrobial activity and protective immunity. In vivo, the absence of STAT3 or STAT6 improved parasite control, while the loss of ROP16 resulted in a marked reduction in parasite numbers and heightened parasite-specific T cell responses. Thus, ROP16 is a virulence factor that can act in cis and trans to promote M2 programs and which limits the magnitude of parasite-specific T cell responses.
- Johnson, H. J., & Koshy, A. A. (2020). Latent Toxoplasmosis Effects on Rodents and Humans: How Much is Real and How Much is Media Hype?. mBio, 11(2). doi:10.1128/mbio.02164-19
- Kochanowsky, J. A., & Koshy, A. A. (2020). A Single Transcription Factor Drives Toxoplasma gondii Differentiation. Cell, 180(2), 216-218.More infoMicrobes that cause persistent infections (e.g., herpes viruses) do so by switching from fast-growing lytic states to slow-growing latent states. Waldman et al. have identified a single transcription factor that governs the switch between the lytic and latent forms of Toxoplasma gondii, a parasite that causes a persistent brain infection.
- McGovern, K. E., Cabral, C. M., Morrison, H. W., & Koshy, A. A. (2020). Aging with Toxoplasma gondii results in pathogen clearance, resolution of inflammation, and minimal consequences to learning and memory. Scientific reports, 10(1), 7979.More infoPersistent inflammation has been identified as a contributor to aging-related neurodegenerative disorders such as Alzheimer's disease. Normal aging, in the absence of dementia, also results in gradual cognitive decline and is thought to arise, in part, because of a chronic pro-inflammatory state in the brain. Toxoplasma gondii is an obligate intracellular parasite that establishes a persistent, asymptomatic infection of the central nervous system (CNS) accompanied by a pro-inflammatory immune response in many of its hosts, including humans and rodents. Several studies have suggested that the inflammation generated by certain strains of T. gondii infection can be neuroprotective in the context of a secondary insult like beta-amyloid accumulation or stroke. Given these neuroprotective studies, we hypothesized that a prolonged infection with T. gondii may protect against age-associated decline in cognition. To test this hypothesis, we infected young adult mice with either of two genetically distinct, persistent T. gondii strains (Prugniaud/type II/haplogroup 2 and CEP/type III/haplogroup 3) and monitored mouse weight, survival, and learning and memory over the ensuing 20 months. At the end of the study, we evaluated CNS inflammation and parasite burden in the surviving mice. We found that parasite infection had no impact on age-associated decline in learning and memory and that by 20 months post infection, in the surviving mice, we found no evidence of parasite DNA, cysts, or inflammation in the CNS. In addition, we found that mice infected with type III parasites, which are supposed to be less virulent than the type II parasites, had a lower rate of long-term survival. Collectively, these data indicate that T. gondii may not cause a life-long CNS infection. Rather, parasites are likely slowly cleared from the CNS and infection and parasite clearance neither positively nor negatively impacts learning and memory in aging.
- Merritt, E. F., Johnson, H. J., Wong, Z. S., Buntzman, A. S., Conklin, A. C., Cabral, C. M., Romanoski, C. E., Boyle, J. P., & Koshy, A. A. (2020). Transcriptional Profiling Suggests T Cells Cluster around Neurons Injected with Toxoplasma gondii Proteins. mSphere, 5(5).More info's tropism for and persistence in the central nervous system (CNS) underlies the symptomatic disease that causes in humans. Our recent work has shown that neurons are the primary CNS cell with which interacts and which it infects This predilection for neurons suggests that 's persistence in the CNS depends specifically upon parasite manipulation of the host neurons. Yet, most work on -host cell interactions has been done and in nonneuronal cells. We address this gap by utilizing our -Cre system that allows permanent marking and tracking of neurons injected with parasite effector proteins Using laser capture microdissection (LCM) and RNA sequencing using RNA-seq, we isolated and transcriptionally profiled -injected neurons (TINs), Bystander neurons (nearby non--injected neurons), and neurons from uninfected mice (controls). These profiles show that TIN transcriptomes significantly differ from the transcriptomes of Bystander and control neurons and that much of this difference is driven by increased levels of transcripts from immune cells, especially CD8 T cells and monocytes. These data suggest that when we used LCM to isolate neurons from infected mice, we also picked up fragments of CD8 T cells and monocytes clustering in extreme proximity around TINs and, to a lesser extent, Bystander neurons. In addition, we found that transcripts were primarily found in the TIN transcriptome, not in the Bystander transcriptome. Collectively, these data suggest that, contrary to common perception, neurons that directly interact with or harbor parasites can be recognized by CD8 T cells. Like other persistent intracellular pathogens, , a protozoan parasite, has evolved to evade the immune system and establish a chronic infection in specific cells and organs, including neurons in the CNS. Understanding 's persistence in neurons holds the potential to identify novel, curative drug targets. The work presented here offers new insights into the neuron- interaction By transcriptionally profiling neurons manipulated by , we unexpectedly revealed that immune cells, and specifically CD8 T cells, appear to cluster around these neurons, suggesting that CD8 T cells specifically recognize parasite-manipulated neurons. Such a possibility supports evidence from other labs that questions the long-standing dogma that neurons are often persistently infected because they are not directly recognized by immune cells such as CD8 T cells. Collectively, these data suggest we reconsider the broader role of neurons in the context of infection and neuroinflammation.
- Tuladhar, S., Kochanowsky, J. A., Bhaskara, A., Ghotmi, Y., Chandrasekaran, S., & Koshy, A. A. (2019). The ROP16III-dependent early immune response determines the subacute CNS immune response and type III Toxoplasma gondii survival. PLoS pathogens, 15(10), e1007856.More infoToxoplasma gondii is an intracellular parasite that persistently infects the CNS and that has genetically distinct strains which provoke different acute immune responses. How differences in the acute immune response affect the CNS immune response is unknown. To address this question, we used two persistent Toxoplasma strains (type II and type III) and examined the CNS immune response at 21 days post infection (dpi). Contrary to acute infection studies, type III-infected mice had higher numbers of total CNS T cells and macrophages/microglia but fewer alternatively activated macrophages (M2s) and regulatory T cells (Tregs) than type II-infected mice. By profiling splenocytes at 5, 10, and 21 dpi, we determined that at 5 dpi type III-infected mice had more M2s while type II-infected mice had more pro-inflammatory macrophages and that these responses flipped over time. To test how these early differences influence the CNS immune response, we engineered the type III strain to lack ROP16 (IIIΔrop16), the polymorphic effector protein that drives the early type III-associated M2 response. IIIΔrop16-infected mice showed a type II-like neuroinflammatory response with fewer infiltrating T cells and macrophages/microglia and more M2s and an unexpectedly low CNS parasite burden. At 5 dpi, IIIΔrop16-infected mice showed a mixed inflammatory response with more pro-inflammatory macrophages, M2s, T effector cells, and Tregs, and decreased rates of infection of peritoneal exudative cells (PECs). These data suggested that type III parasites need the early ROP16-associated M2 response to avoid clearance, possibly by the Immunity-Related GTPases (IRGs), which are IFN-γ- dependent proteins essential for murine defenses against Toxoplasma. To test this possibility, we infected IRG-deficient mice and found that IIIΔrop16 parasites now maintained parental levels of PECs infection. Collectively, these studies suggest that, for the type III strain, rop16III plays a key role in parasite persistence and influences the subacute CNS immune response.
- Kochanowsky, J. A., & Koshy, A. A. (2018). Toxoplasma gondii. Curr Bio.
- Kochanowsky, J. A., & Koshy, A. A. (2018). Toxoplasma gondii. Current biology : CB, 28(14), R770-R771.More infoPutting cat lovers on notice: Toxoplasma gondii is here to control your brain…or is it? Kochanowsky and Koshy shine the spotlight on this notorious pathogen.
- Mendez, O. A., Potter, C. J., Valdez, M. A., Bello, T., Trouard, T. P., & Koshy, A. A. (2018). Semi-automated quantification and neuroanatomical mapping of heterogeneous cell populations.. Journal of neuroscience methods, 305, 98-104. doi:10.1016/j.jneumeth.2018.05.008More infoOur group studies the interactions between cells of the brain and the neurotropic parasite Toxoplasma gondii. Using an in vivo system that allows us to permanently mark and identify brain cells injected with Toxoplasma protein, we have identified that Toxoplasma-injected neurons (TINs) are heterogeneously distributed throughout the brain. Unfortunately, standard methods to quantify and map heterogeneous cell populations onto a reference brain atlas are time consuming and prone to user bias..We developed a novel MATLAB-based semi-automated quantification and mapping program to allow the rapid and consistent mapping of heterogeneously distributed cells on to the Allen Institute Mouse Brain Atlas. The system uses two-threshold background subtraction to identify and quantify cells of interest..We demonstrate that we reliably quantify and neuroanatomically localize TINs with low intra- or inter-observer variability. In a follow up experiment, we show that specific regions of the mouse brain are enriched with TINs..The procedure we use takes advantage of simple immunohistochemistry labeling techniques, use of a standard microscope with a motorized stage, and low cost computing that can be readily obtained at a research institute. To our knowledge there is no other program that uses such readily available techniques and equipment for mapping heterogeneous populations of cells across the whole mouse brain..The quantification method described here allows reliable visualization, quantification, and mapping of heterogeneous cell populations in immunolabeled sections across whole mouse brains.
- Cabral, C. M., McGovern, K. E., Franco, J. B., MacDonald, W. R., & Koshy, A. A. (2017). Understanding the strain-specific effects of Toxoplasma gondii on amyloid-beta deposition. ASN Neuro.
- Cabral, C. M., McGovern, K. E., MacDonald, W. R., Franco, J., & Koshy, A. A. (2017). Dissecting Amyloid Beta Deposition Using Distinct Strains of the Neurotropic Parasite Toxoplasma gondii as a Novel Tool. ASN neuro, 9(4), 1759091417724915.More infoGenetic and pathologic data suggest that amyloid beta (Aβ), produced by processing of the amyloid precursor protein, is a major initiator of Alzheimer's disease (AD). To gain new insights into Aβ modulation, we sought to harness the power of the coevolution between the neurotropic parasite Toxoplasma gondii and the mammalian brain. Two prior studies attributed Toxoplasma-associated protection against Aβ to increases in anti-inflammatory cytokines (TGF-β and IL-10) and infiltrating phagocytic monocytes. These studies only used one Toxoplasma strain making it difficult to determine if the noted changes were associated with Aβ protection or simply infection. To address this limitation, we infected a third human amyloid precursor protein AD mouse model (J20) with each of the genetically distinct, canonical strains of Toxoplasma (Type I, Type II, or Type III). We then evaluated the central nervous system (CNS) for Aβ deposition, immune cell responses, global cytokine environment, and parasite burden. We found that only Type II infection was protective against Aβ deposition despite both Type II and Type III strains establishing a chronic CNS infection and inflammatory response. Compared with uninfected and Type I-infected mice, both Type II- and Type III-infected mice showed increased numbers of CNS T cells and microglia and elevated pro-inflammatory cytokines, but neither group showed a >2-fold elevation of TGF-β or IL-10. These data suggest that we can now use our identification of protective (Type II) and nonprotective (Type III) Toxoplasma strains to determine what parasite and host factors are linked to decreased Aβ burden rather than simply with infection.
- Mendez, O. A., & Koshy, A. A. (2017). Toxoplasma gondii: Entry, association, and physiologic influences on the central nervous system. Plos Pathogens.
- Mendez, O. A., & Koshy, A. A. (2017). Toxoplasma gondii: Entry, association, and physiological influence on the central nervous system. PLoS pathogens, 13(7), e1006351.More infoToxoplasma gondii is one of the world's most successful parasites, in part because of its ability to infect and persist in most warm-blooded animals. A unique characteristic of T. gondii is its ability to persist in the central nervous system (CNS) of a variety of hosts, including humans and rodents. How, what, and why T. gondii encysts in the CNS has been the topic of study for decades. In this review, we will discuss recent work on how T. gondii is able to traverse the unique barrier surrounding the CNS, what cells of the CNS play host to T. gondii, and finally, how T. gondii infection may influence global and cellular physiology of the CNS.
- Cabral, C. M., Tuladhar, S., Dietrich, H. K., Nguyen, E., MacDonald, W. R., Trivedi, T., Devineni, A., & Koshy, A. A. (2016). Neurons are the primary target cell for the brain-tropic intracellular parasite Toxoplasma gondii.. PLoS Pathogens.
- Hidano, S., Randall, L. M., Dawson, L., Dietrich, H. K., Konradt, C., Klover, P. J., John, B., Harris, T. H., Fang, Q., Turek, B., Kobayashi, T., Hennighausen, L., Beiting, D. P., Koshy, A. A., & Hunter, C. A. (2016). STAT1 Signaling in Astrocytes Is Essential for Control of Infection in the Central Nervous System. mBio, 7(6).More infoThe local production of gamma interferon (IFN-γ) is important to control Toxoplasma gondii in the brain, but the basis for these protective effects is not fully understood. The studies presented here reveal that the ability of IFN-γ to inhibit parasite replication in astrocytes in vitro is dependent on signal transducer and activator of transcription 1 (STAT1) and that mice that specifically lack STAT1 in astrocytes are unable to limit parasite replication in the central nervous system (CNS). This susceptibility is associated with a loss of antimicrobial pathways and increased cyst formation in astrocytes. These results identify a critical role for astrocytes in limiting the replication of an important opportunistic pathogen.
- Konradt, C., Ueno, N., Christian, D. A., DeLong, J., Harms-Pritchard, G., Herz, J., Bzik, D. J., McGavern, D. B., Koshy, A. A., Lodoen, M. B., & Hunter, C. A. (2016). Endothelial cells as a replicative niche for pathway entry to the CNS. Nature Microbiology.
- Koshy, A. A. (2015). Review of Infections of the Central Nervous System. JAMA Neurology, 72(8), 951-951. doi:10.1001/jamaneurol.2015.0967
- Blader, I. J., & Koshy, A. A. (2014). Toxoplasma gondii development of its replicative niche: in its host cell and beyond. Eukaryotic cell, 13(8), 965-76.More infoIntracellular pathogens can replicate efficiently only after they manipulate and modify their host cells to create an environment conducive to replication. While diverse cellular pathways are targeted by different pathogens, metabolism, membrane and cytoskeletal architecture formation, and cell death are the three primary cellular processes that are modified by infections. Toxoplasma gondii is an obligate intracellular protozoan that infects ∼30% of the world's population and causes severe and life-threatening disease in developing fetuses, in immune-comprised patients, and in certain otherwise healthy individuals who are primarily found in South America. The high prevalence of Toxoplasma in humans is in large part a result of its ability to modulate these three host cell processes. Here, we highlight recent work defining the mechanisms by which Toxoplasma interacts with these processes. In addition, we hypothesize why some processes are modified not only in the infected host cell but also in neighboring uninfected cells.
- Cekanaviciute, E., Dietrich, H. K., Axtell, R. C., Williams, A. M., Egusquiza, R., Wai, K. M., Koshy, A. A., & Buckwalter, M. S. (2014). Astrocytic TGF-β signaling limits inflammation and reduces neuronal damage during central nervous system Toxoplasma infection. Journal of immunology (Baltimore, Md. : 1950), 193(1), 139-49.More infoThe balance between controlling infection and limiting inflammation is particularly precarious in the brain because of its unique vulnerability to the toxic effects of inflammation. Astrocytes have been implicated as key regulators of neuroinflammation in CNS infections, including infection with Toxoplasma gondii, a protozoan parasite that naturally establishes a chronic CNS infection in mice and humans. In CNS toxoplasmosis, astrocytes are critical to controlling parasite growth. They secrete proinflammatory cytokines and physically encircle parasites. However, the molecular mechanisms used by astrocytes to limit neuroinflammation during toxoplasmic encephalitis have not yet been identified. TGF-β signaling in astrocytes is of particular interest because TGF-β is universally upregulated during CNS infection and serves master regulatory and primarily anti-inflammatory functions. We report in this study that TGF-β signaling is activated in astrocytes during toxoplasmic encephalitis and that inhibition of astrocytic TGF-β signaling increases immune cell infiltration, uncouples proinflammatory cytokine and chemokine production from CNS parasite burden, and increases neuronal injury. Remarkably, we show that the effects of inhibiting astrocytic TGF-β signaling are independent of parasite burden and the ability of GFAP(+) astrocytes to physically encircle parasites.
- Christian, D. A., Koshy, A. A., Reuter, M. A., Betts, M. R., Boothroyd, J. C., & Hunter, C. A. (2014). Use of transgenic parasites and host reporters to dissect events that promote interleukin-12 production during toxoplasmosis. Infection and immunity, 82(10), 4056-67.More infoThe intracellular parasite Toxoplasma gondii has multiple strategies to alter host cell function, including the injection of rhoptry proteins into the cytosol of host cells as well as bystander populations, but the consequence of these events is unclear. Here, a reporter system using fluorescent parasite strains that inject Cre recombinase with their rhoptry proteins (Toxoplasma-Cre) was combined with Ai6 Cre reporter mice to identify cells that have been productively infected, that have been rhoptry injected but lack the parasite, or that have phagocytosed T. gondii. The ability to distinguish these host-parasite interactions was then utilized to dissect the events that lead to the production of interleukin-12 p40 (IL-12p40), which is required for resistance to T. gondii. In vivo, the use of invasion-competent or invasion-inhibited (phagocytosed) parasites with IL-12p40 (YET40) reporter mice revealed that dendritic cell (DC) and macrophage populations that phagocytose the parasite or are infected can express IL-12p40 but are not the major source, as larger numbers of uninfected cells secrete this cytokine. Similarly, the use of Toxoplasma-Cre parasite strains indicated that dendritic cells and inflammatory monocytes untouched by the parasite and not cells injected by the parasite are the primary source of IL-12p40. These results imply that a soluble host or parasite factor is responsible for the bulk of IL-12p40 production in vivo, rather than cellular interactions with T. gondii that result in infection, infection and clearance, injection of rhoptry proteins, or phagocytosis of the parasite.
- Dupont, C. D., Christian, D. A., Selleck, E. M., Pepper, M., Leney-Greene, M., Harms Pritchard, G., Koshy, A. A., Wagage, S., Reuter, M. A., Sibley, L. D., Betts, M. R., & Hunter, C. A. (2014). Parasite fate and involvement of infected cells in the induction of CD4+ and CD8+ T cell responses to Toxoplasma gondii. PLoS pathogens, 10(4), e1004047.More infoDuring infection with the intracellular parasite Toxoplasma gondii, the presentation of parasite-derived antigens to CD4+ and CD8+ T cells is essential for long-term resistance to this pathogen. Fundamental questions remain regarding the roles of phagocytosis and active invasion in the events that lead to the processing and presentation of parasite antigens. To understand the most proximal events in this process, an attenuated non-replicating strain of T. gondii (the cpsII strain) was combined with a cytometry-based approach to distinguish active invasion from phagocytic uptake. In vivo studies revealed that T. gondii disproportionately infected dendritic cells and macrophages, and that infected dendritic cells and macrophages displayed an activated phenotype characterized by enhanced levels of CD86 compared to cells that had phagocytosed the parasite, thus suggesting a role for these cells in priming naïve T cells. Indeed, dendritic cells were required for optimal CD4+ and CD8+ T cell responses, and the phagocytosis of heat-killed or invasion-blocked parasites was not sufficient to induce T cell responses. Rather, the selective transfer of cpsII-infected dendritic cells or macrophages (but not those that had phagocytosed the parasite) to naïve mice potently induced CD4+ and CD8+ T cell responses, and conferred protection against challenge with virulent T. gondii. Collectively, these results point toward a critical role for actively infected host cells in initiating T. gondii-specific CD4+ and CD8+ T cell responses.
- Han, S., Melichar, H. J., Coombes, J. L., Chan, S. W., Koshy, A. A., Boothroyd, J. C., Barton, G. M., & Robey, E. A. (2014). Internalization and TLR-dependent type I interferon production by monocytes in response to Toxoplasma gondii. Immunology and cell biology, 92(10), 872-81.More infoThe classic anti-viral cytokine interferon (IFN)-β can be induced during parasitic infection, but relatively little is know about the cell types and signaling pathways involved. Here we show that inflammatory monocytes (IMs), but not neutrophils, produce IFN-β in response to T. gondii infection. This difference correlated with the mode of parasite entry into host cells, with phagocytic uptake predominating in IMs and active invasion predominating in neutrophils. We also show that expression of IFN-β requires phagocytic uptake of the parasite by IMs, and signaling through Toll-like receptors (TLRs) and MyD88. Finally, we show that IMs are major producers of IFN-β in mesenteric lymph nodes following in vivo oral infection of mice, and mice lacking the receptor for type I IFN-1 show higher parasite loads and reduced survival. Our data reveal a TLR and internalization-dependent pathway in IMs for IFN-β induction to a non-viral pathogen.
- Koshy, A. A., & Cabral, C. M. (2014). 3-D imaging and analysis of neurons infected in vivo with Toxoplasma gondii. Journal of visualized experiments : JoVE.More infoToxoplasma gondii is an obligate, intracellular parasite with a broad host range, including humans and rodents. In both humans and rodents, Toxoplasma establishes a lifelong persistent infection in the brain. While this brain infection is asymptomatic in most immunocompetent people, in the developing fetus or immunocompromised individuals such as acquired immune deficiency syndrome (AIDS) patients, this predilection for and persistence in the brain can lead to devastating neurologic disease. Thus, it is clear that the brain-Toxoplasma interaction is critical to the symptomatic disease produced by Toxoplasma, yet we have little understanding of the cellular or molecular interaction between cells of the central nervous system (CNS) and the parasite. In the mouse model of CNS toxoplasmosis it has been known for over 30 years that neurons are the cells in which the parasite persists, but little information is available about which part of the neuron is generally infected (soma, dendrite, axon) and if this cellular relationship changes between strains. In part, this lack is secondary to the difficulty of imaging and visualizing whole infected neurons from an animal. Such images would typically require serial sectioning and stitching of tissue imaged by electron microscopy or confocal microscopy after immunostaining. By combining several techniques, the method described here enables the use of thick sections (160 µm) to identify and image whole cells that contain cysts, allowing three-dimensional visualization and analysis of individual, chronically infected neurons without the need for immunostaining, electron microscopy, or serial sectioning and stitching. Using this technique, we can begin to understand the cellular relationship between the parasite and the infected neuron.
- Lopez, J., Lomen-Hoerth, C., Deutsch, G. K., Kerchner, G. A., & Koshy, A. (2014). Influenza-associated global amnesia and hippocampal imaging abnormality. Neurocase, 20(4), 446-51.More infoThe acute phase of influenza infection is rarely associated with significant cognitive dysfunction. We describe a case of a 24 year-old man who developed global amnesia in the acute phase of influenza A infection. His deficits resolved over the course of several weeks. Transient abnormalities of diffusion and T2-weighted imaging were seen in the bilateral hippocampi. We review cerebral complications of influenza and discuss the possible role of previously proposed mechanisms in our patient's case.
- Caffaro, C. E., Koshy, A. A., Liu, L., Zeiner, G. M., Hirschberg, C. B., & Boothroyd, J. C. (2013). A nucleotide sugar transporter involved in glycosylation of the Toxoplasma tissue cyst wall is required for efficient persistence of bradyzoites. PLoS pathogens, 9(5), e1003331.More infoToxoplasma gondii is an intracellular parasite that transitions from acute infection to a chronic infective state in its intermediate host via encystation, which enables the parasite to evade immune detection and clearance. It is widely accepted that the tissue cyst perimeter is highly and specifically decorated with glycan modifications; however, the role of these modifications in the establishment and persistence of chronic infection has not been investigated. Here we identify and biochemically and biologically characterize a Toxoplasma nucleotide-sugar transporter (TgNST1) that is required for cyst wall glycosylation. Toxoplasma strains deleted for the TgNST1 gene (Δnst1) form cyst-like structures in vitro but no longer interact with lectins, suggesting that Δnst1 strains are deficient in the transport and use of sugars for the biosynthesis of cyst-wall structures. In vivo infection experiments demonstrate that the lack of TgNST1 activity does not detectably impact the acute (tachyzoite) stages of an infection or tropism of the parasite for the brain but that Δnst1 parasites are severely defective in persistence during the chronic stages of the infection. These results demonstrate for the first time the critical role of parasite glycoconjugates in the persistence of Toxoplasma tissue cysts.
- Coombes, J. L., Charsar, B. A., Han, S., Halkias, J., Chan, S. W., Koshy, A. A., Striepen, B., & Robey, E. A. (2013). Motile invaded neutrophils in the small intestine of Toxoplasma gondii-infected mice reveal a potential mechanism for parasite spread. Proceedings of the National Academy of Sciences of the United States of America, 110(21), E1913-22.More infoToxoplasma gondii infection occurs through the oral route, but we lack important information about how the parasite interacts with the host immune system in the intestine. We used two-photon laser-scanning microscopy in conjunction with a mouse model of oral T. gondii infection to address this issue. T. gondii established discrete foci of infection in the small intestine, eliciting the recruitment and transepithelial migration of neutrophils and inflammatory monocytes. Neutrophils accounted for a high proportion of actively invaded cells, and we provide evidence for a role for transmigrating neutrophils and other immune cells in the spread of T. gondii infection through the lumen of the intestine. Our data identify neutrophils as motile reservoirs of T. gondii infection and suggest a surprising retrograde pathway for parasite spread in the intestine.
- Koshy, A. A., Dietrich, H. K., Christian, D. A., Melehani, J. H., Shastri, A. J., Hunter, C. A., & Boothroyd, J. C. (2012). Toxoplasma co-opts host cells it does not invade. PLoS pathogens, 8(7), e1002825.More infoLike many intracellular microbes, the protozoan parasite Toxoplasma gondii injects effector proteins into cells it invades. One group of these effector proteins is injected from specialized organelles called the rhoptries, which have previously been described to discharge their contents only during successful invasion of a host cell. In this report, using several reporter systems, we show that in vitro the parasite injects rhoptry proteins into cells it does not productively invade and that the rhoptry effector proteins can manipulate the uninfected cell in a similar manner to infected cells. In addition, as one of the reporter systems uses a rhoptry:Cre recombinase fusion protein, we show that in Cre-reporter mice infected with an encysting Toxoplasma-Cre strain, uninfected-injected cells, which could be derived from aborted invasion or cell-intrinsic killing after invasion, are actually more common than infected-injected cells, especially in the mouse brain, where Toxoplasma encysts and persists. This phenomenon has important implications for how Toxoplasma globally affects its host and opens a new avenue for how other intracellular microbes may similarly manipulate the host environment at large.
- Koshy, A. A., Fouts, A. E., Lodoen, M. B., Alkan, O., Blau, H. M., & Boothroyd, J. C. (2010). Toxoplasma secreting Cre recombinase for analysis of host-parasite interactions.. Nature methods, 7(4), 307-9. doi:10.1038/nmeth.1438More infoWe describe a Toxoplasma gondii strain that will permit the use of site-specific recombination to study the host-parasite interactions of this organism. This Toxoplasma strain efficiently injects a Cre fusion protein into host cells. In a Cre-reporter cell line, a single parasite invasion induced Cre-mediated recombination in 95% of infected host cells. By infecting Cre-reporter mice with these parasites, we also monitored host-cell infection in vivo.
- Chiu, C. Y., Rouskin, S., Koshy, A., Urisman, A., Fischer, K. F., Yagi, S., Schnurr, D. P., Eckburg, P. B., Tompkins, L. S., Blackburn, B. G., Merker, J. D., Patterson, B. K., Ganem, D., & Derisi, J. L. (2006). Microarray detection of human parainfluenzavirus 4 infection associated with respiratory failure in an immunocompetent adult.. Clinical infectious diseases : an official publication of the Infectious Diseases Society of America, 43(8), e71-6. doi:10.1086/507896More infoA pan-viral DNA microarray, the Virochip (University of California, San Francisco), was used to detect human parainfluenzavirus 4 (HPIV-4) infection in an immunocompetent adult presenting with a life-threatening acute respiratory illness. The virus was identified in an endotracheal aspirate specimen, and the microarray results were confirmed by specific polymerase chain reaction and serological analysis for HPIV-4. Conventional clinical laboratory testing using an extensive panel of microbiological tests failed to yield a diagnosis. This case suggests that the potential severity of disease caused by HPIV-4 in adults may be greater than previously appreciated and illustrates the clinical utility of a microarray for broad-based viral pathogen screening.
- Jiang, X., Mu, D., Manabat, C., Koshy, A. A., Christen, S., Tauber, M. G., Vexler, Z. S., & Ferriero, D. M. (2004). Differential vulnerability of immature murine neurons to oxygen-glucose deprivation.. Experimental neurology, 190(1), 224-32. doi:10.1016/j.expneurol.2004.07.010More infoIn vivo studies support selective neuronal vulnerability to hypoxia-ischemia (HI) in the developing brain. Since differences in intrinsic properties of neurons might be responsible, pure cultures containing immature neurons (6-8 days in vitro) isolated from mouse cortex and hippocampus, regions chosen for their marked vulnerability to oxidative stress, were studied under in vitro ischemic conditions-oxygen-glucose deprivation (OGD). Twenty-four hours of reoxygenation after 2.5 h of OGD induced significantly greater cell death in hippocampal than in cortical neurons (67.8% vs. 33.4%, P = 0.0068). The expression of neuronal nitric oxide synthase (nNOS) protein, production of nitric oxide (NO), and reactive oxygen species (ROS), as well as glutathione peroxidase (GPx) activity and intracellular levels of reduced glutathione (GSH), were measured as indicators of oxidative stress. Hippocampal neurons had markedly higher nNOS expression than cortical neurons by 24 h of reoxygenation, which coincided with an increase in NO production, and significantly greater ROS accumulation. GPx activity declined significantly in hippocampal but not in cortical neurons at 4 and 24 h after OGD. The decrease in GSH level in hippocampal neurons correlated with the decline of GPx activity. Our data suggest that developing hippocampal neurons are more sensitive to OGD than cortical neurons. This finding supports our in vivo studies showing that mouse hippocampus is more vulnerable than cortex after neonatal HI. An imbalance between excess prooxidant production (increased nNOS expression, and NO and ROS production) and insufficient antioxidant defenses created by reduced GPx activity and GSH levels may, in part, explain the higher susceptibility to OGD of immature hippocampal neurons.
- Almli, L. M., Hamrick, S. E., Koshy, A. A., Tauber, M. G., & Ferriero, D. M. (2001). Multiple pathways of neuroprotection against oxidative stress and excitotoxic injury in immature primary hippocampal neurons.. Brain research. Developmental brain research, 132(2), 121-9. doi:10.1016/s0165-3806(01)00302-9More infoIn the immature brain hydrogen peroxide accumulates after excitotoxic hypoxia-ischemia and is neurotoxic. Immature hippocampal neurons were exposed to N-methyl-D-aspartate (NMDA), a glutamate agonist, and hydrogen peroxide (H(2)O(2)) and the effects of free radical scavenging and transition metal chelation on neurotoxicity were studied. alpha-Phenyl-N-tert.-butylnitrone (PBN), a known superoxide scavenger, attenuated both H(2)O(2) and NMDA mediated toxicity. Treatment with desferrioxamine (DFX), an iron chelator, at the time of exposure to H(2)O(2) was ineffective, but pretreatment was protective. DFX also protected against NMDA toxicity. TPEN, a metal chelator with higher affinities for a broad spectrum of transition metal ions, also protected against H(2)O(2) toxicity but was ineffective against NMDA induced toxicity. These data suggest that during exposure to free radical and glutamate agonists, the presence of iron and other free metal ions contribute to neuronal cell death. In the immature nervous system this neuronal injury can be attenuated by free radical scavengers and metal chelators.
Presentations
- Koshy, A. A. (2020, August). CNS Parasites: who, when, where, and how to diagnose and treat. Seminar Series. Tucson, Arizona: Dept of Neurology, University of Arizona.
- Koshy, A. A. (2020, January). Re-defining Toxoplasma gondii-neuron interactions. Seminar Series. Stanford, CA: Department of Microbiology & Immunology, Stanford University.
- Koshy, A. A. (2020, March). Re-defining Toxoplasma gondii-neuron interactions. 15th Annual Frontiers in Immunobiology & Immunopathogenesis Symposium. Tucson, AZ: Department of Immunobiology, University of Arizona.
- Koshy, A. A. (2020, March). Re-defining Toxoplasma gondii-neuron interactions. Seminar Series. Athens, GA: Department of Cellular Biology, University of Georgia, Athens (UGA).
- Koshy, A. A. (2021, January). CNS Infections and COVID Associations. Seminar Series. Tucson, Arizona: Division of Infectious Diseases, Dept of Medicine, University of Arizona.
- Koshy, A. A. (2019, July). Interrogating CNS-T. gondii interactions at the single cell level in vivo. Microbial Pathogenesis Conference (Invited talk). Snowmass, Co: FASEB.
- Koshy, A. A. (2019, June). “CNS toxoplasmosis: friend or foe?”. State-of-the-Art Lecture (Neurology). Phoenix, AZ: ACVIM (Annual Meeting).
- Koshy, A. A. (2019, November). The Toxoplasma-neuron interface: challenging our understanding of neuron capabilities. ACMCIP (American Committee of Molecular, Cellular and Immunoparasitology) Symposium (Invited talk). National Harbor, MD: ASTMH (American Society of Tropical Medicine and Hygiene).
- Koshy, A. A. (2019, Sept 5). CNS Parasites: who, when, where, and how to diagnose and treat. Seminar Series. Tucson, Arizona: Div of Infectious Disease, Dept of Medicine, University of Arizona.
- Koshy, A. A. (2018, April). “CNS toxoplasmosis- redefining our model of brain infections”. Bi-annual Graduate Program Symposium. Seattle, WA: Molecular & Cellular Biology Program, University of Washington.
- Koshy, A. A. (2018, April). “Defining the drivers of CNS immune responses to Toxoplasma gondii”. Seminar Series. Madison, WI: Medical Microbiology & Immunology, University of Wisconsin.
- Koshy, A. A. (2018, April). “Interrogating Toxoplasma-neuron interactions in vivo”. Seminar Series. Madison, WI: Department of Pathology, University of Wisconsin.
- Koshy, A. A. (2018, Dec). Toxoplasma gondii-neuron interactions: new insights into CNS infections. Seminar Series. San Francisco, CA: University of California, San Francisco.
- Koshy, A. A. (2018, June). Neuronal defense mechanisms against Toxoplasma gondii infection. Conference. Newport, RI: GRC: Biology of Host-Parasite Interactions.
- Koshy, A. A. (2018, March). Defining Toxoplasma-neuron interactions at the single cell level in vivo. Conference. Riverside, CA: American Society for Neurochemistry Annual Meeting.
- Koshy, A. A. (2018, Sept). “CNS toxoplasmosis: friend or foe?”. Seminar Series. South San Francisco, CA: Denali Therapeutics.
- Koshy, A. A. (2017, February). Interrogating CNS-Toxoplasma interactions in vivo. Seminar Series. Tucson, Arizona: Department of Pharmacology, University of Arizona.
- Koshy, A. A. (2017, July 12). Studying the brain-Toxoplasma gondii interface. Seminar Series. Tucson, Arizona: KEYS program, BIO5.
- Koshy, A. A. (2017, June). Toxoplasma gondii-neuron interactions in vivo: a transcriptomics approach.. Conference. Tomar, Portugal.: 14th International Congress on Toxoplasmosis and Toxoplasma gondii..
- Koshy, A. A. (2017, Mar 9). Defining the neuroprotective effect of chronic toxoplasmosis. Conference. Phoenix, AZ: 2nd Annual ABRC Research Conference.
- Koshy, A. A. (2017, Nov 3). Toxoplasma-neuron interactions in vivo. Conference. Tempe, AZ: ASU-UA Virology Symposium.
- Koshy, A. A. (2017, Sept 5). CNS Parasites: who, when, where, and how to diagnose and treat. Seminar Series. Tucson, Arizona: Div of Infectious Disease, Dept of Medicine, University of Arizona.
- Koshy, A. A. (2017, September). Understanding the Toxoplasma-neuron interaction at the cellular and molecular level. Seminar Series. Indianapolis, IN: Research Seminar Series, Dept of Pharmacology & Toxicology, School of Medicine, Indiana University.
- Koshy, A. A. (2016, Apr 25). Interrogating the CNS-Toxoplasma interaction in vivo. Seminar Series. Pittsburgh, PA: Department of Biological Sciences, University of Pittsburgh.
- Koshy, A. A. (2016, Mar 28). Interrogating the CNS-Toxoplasma interaction in vivo. Seminar Series. Hanover, NH: Department of Microbiology and Molecular Pathogenesis Program, Dartmouth University.
- Koshy, A. A. (2016, Mar 8). Using the Cre/loxP system to understand brain-parasite interactions. Conference. Tucson, Arizona: Neuroscience Datablitz, International Mendel Day, University of Arizona.
- Koshy, A. A. (2016, Oct 13). Toxoplasma gondii & the CNS: Frenemies- the crossroads of microbiology, immunology, & neuroscience. Seminar Series. Tucson, Arizona: Research Seminar Series, Department of Medicine, University of Arizona.
- Koshy, A. A. (2016, Oct 2). Toxoplasma gondii’s preference for neurons: location, location, location. Seminar Series. Philadelphia, PA: School of Veterinary Medicine, University of Pennsylvania.
- Koshy, A. A. (2015, Apr 21). Can a common brain parasite help find novel therapeutic targets for AD?. Seminar Series. Sun City, AZ: Banner Sun Health Research Institute.
- Koshy, A. A. (2015, Jul 24). Toxoplasma gondii & the CNS: Frenemies?. Seminar Series. Tucson, Arizona: Department of Neurology, University of Arizona.
- Koshy, A. A. (2015, Jun 12). Toxoplasma gondii & the CNS: Frenemies?. Seminar Series. Stanford, CA: Department of Neurology, Stanford University.
- Koshy, A. A. (2015, Mar 25). Using Toxoplasma gondii to understand brain immune capabilities. Seminar Series. Dallas, Texas: Department of Molecular Biology & Biochemistry,UC Irvine.
- Koshy, A. A. (2015, Sept 9). Redefining the CNS-Toxoplasma interaction. Seminar Series. Washington, DC: Department of Microbiology, Immunology & Tropical Medicine, George Washington University.
- Koshy, A. A. (2014, Nov 20). Changing the parasitic game: the new in vivo Toxoplasma-Cre model. Seminar. Irvine, CA: Department of Molecular Biology & Biochemistry,UC Irvine.
- Koshy, A. A. (2014, Oct 29). Toxoplasma-Cre: a new understanding of CNS-Toxoplasma interactions. Seminar Series. Tucson, Arizona: Dept of Cellular and Molecular Medicine; Dept of Molecular & Cellular Biology; Dept of Chemistry Biochemistry.
Poster Presentations
- Koshy, A. A. (2017, October). Determining how different Toxoplasma strains drive strain-specific CNS-immune response. Conference. San Diego, CA.: 142nd American Neurological Association Annual Meeting..
Reviews
- Johnson, H. J., & Koshy, A. A. (2020. Latent Toxoplasmosis Effects on Rodents and Humans: How Much is Real and How Much is Media Hype?.More infois a ubiquitous, intracellular protozoan parasite with a broad range of intermediate hosts, including humans and rodents. In many hosts, establishes a latent long-term infection by converting from its rapidly dividing or lytic form to its slowly replicating and encysting form. In humans and rodents, the major organ for encystment is the central nervous system (CNS), which has led many to investigate how this persistent CNS infection might influence rodent and human behavior and, more recently, neurodegenerative diseases. Given the interest in this topic, here we seek to take a global approach to the data for and against the effects of latent on behavior and neurodegeneration and the proposed mechanisms that might underlie behavior modifications.