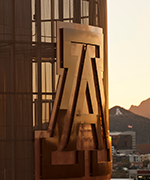
Trent R Anderson
- Associate Professor, Pharmacology
- Member of the Graduate Faculty
- Associate Professor, Neuroscience - GIDP
- UA Associate, Research
- (602) 827-2158
- Life Sciences North, Rm. 660
- Tucson, AZ 85724
- andersot@arizona.edu
Biography
The brain is a fascinating lesson in duality – constantly balancing between the needs for diametrically opposed extremes. At its core it is an elegantly simplistic processing and computation center and yet its function results in the complicated behaviour, perception and reality we all experience. Built from simple single neurons the brain forms emergent networks that drive and govern our basic behaviour and how we perceive and interpret the world around us. Specifically, as a scientist I am intrigued by the duality of the balance the brain strikes between excitation and inhibition – tempering the need for excitatory activity to convey sensory and motor information with inhibitory activity that prevents run away excitation. Understanding the regulatory mechanisms that control this balance as well as the way disease states alter this balance has been the focus of my research career.
Did you know the brain consumes over 25% of the energy the body produces? This rate of consumption is 10 times greater than that of any other tissue. What is intriguing is given this extraordinary demand for energy the brain functions “inefficiently” – its excitatory activity is constantly being suppressed by an over-riding inhibitory activity. It is as if the brain is applying pressure to both the gas pedal and brake at the same time. The necessity for this inhibitory action is clearly evident as its loss or reduction has profound implication on the development and propagation of numerous disease states including epilepsy, Parkinson’s disease, stroke and migraine. In the cerebral cortex, the higher order processing center of the brain, inhibitory interneurons are responsible for this “brake”. These interneurons are a distinct and yet diverse group of specialized neurons with varying anatomical, pharmacologic and physiological properties that make them ideally situated to understand and manipulate this balance.
My research program combines aspects of cellular, synaptic and network neuroscience by using advanced tools in cellular physiology, neuropharmacology, imaging and molecular biology to elucidate mechanisms regulating excitability. Ongoing research points to neurosteroids as a prime candidate in regulating the balance between excitation and inhibition. Cortical neurons possess unique properties and sensitivities to neurosteroids that may be exploited to increase our understanding of the way the brain functions and as potential sources of therapeutic action. Steroids such as progesterone, pregnenonlone and dehydropiandrosterone are normally associated with their peripheral origin and action but have recently been shown to be de-novo synthesized in the brain itself. Specifically, several reports have indicated the ability of neurosteroids to alter both inhibitory (GABA) and excitatory (NMDA) function. Determining selective and novel pathways to up or down regulate the excitability of the brain may reveal significant sources of new therapeutic potential.
Degrees
- Ph.D. Anatomy and Cell Biology
- Queen's University
- B.Sc.H Life Sciences
- Queens University, Kingston, Ontario
Work Experience
- Stanford University (2005 - 2008)
- University of Calgary - Hotchkiss Brain Institute (2002 - 2005)
Interests
Research
Migraine, Epilepy, Traumatic Brain Injury, Electrophsyiology, Optogenetics, Imaging, Cancer; Neuro-degenerative, developmental and psychiatric disease; Developmental, cell and molecular biology; Signaling and steroid biology
Teaching
Anatomy, Neuroscience, Neuroanatomy, Neurophysiology, Neuroanatomy
Courses
2024-25 Courses
-
Directed Research
ABBS 792 (Spring 2025) -
Directed Research
PHCL 692 (Spring 2025) -
Dissertation
PCOL 920 (Spring 2025) -
Methods In Neuroscience
NRSC 700 (Spring 2025) -
Research
PHCL 900 (Spring 2025) -
Research Conference
PCOL 695A (Spring 2025) -
Directed Research
PHCL 692 (Fall 2024) -
Pharm of Cardio,Pulm,GI&CNS
PHCL 601C (Fall 2024) -
Pharmacology: Gen. Principles
PHCL 601A (Fall 2024) -
Research
PHCL 900 (Fall 2024) -
Research Conference
PCOL 695A (Fall 2024)
2023-24 Courses
-
Directed Research
PHCL 692 (Spring 2024) -
Research
PHCL 900 (Spring 2024) -
Research
PHSC 900 (Spring 2024) -
Research Conference
PCOL 695A (Spring 2024) -
Directed Research
PHCL 692 (Fall 2023) -
Pharmacology: Gen. Principles
PHCL 601A (Fall 2023) -
Research
PHCL 900 (Fall 2023)
2022-23 Courses
-
Directed Research
MCB 792 (Spring 2023) -
Directed Research
PHSC 792A (Spring 2023) -
Pharm of Cardio,Pulm,GI&CNS
PHCL 601C (Fall 2022) -
Pharmacology: Gen. Principles
PHCL 601A (Fall 2022)
2021-22 Courses
-
Cellular Molecular& Neural Bio
CTS 555 (Fall 2021)
2020-21 Courses
-
Cellular Molecular& Neural Bio
CTS 555 (Fall 2020)
2019-20 Courses
-
Cellular Molecular& Neural Bio
CTS 555 (Fall 2019)
Scholarly Contributions
Journals/Publications
- Knowles, S. J., Holter, M. C., Li, G., Bjorklund, G. R., Rees, K. P., Martinez-Fuentes, J. S., Nishimura, K. J., Afshari, A. E., Fry, N., Stafford, A. M., Vogt, D., Mangone, M., Anderson, T., & Newbern, J. M. (2023). Multifunctional requirements for ERK1/2 signaling in the development of ganglionic eminence derived glia and cortical inhibitory neurons. eLife. doi:10.7554/elife.88313
- Rudolph, M., Kopruszinski, C., Wu, C., Navratilova, E., Schwedt, T. J., Dodick, D. W., Porreca, F., & Anderson, T. (2023). Identification of brain areas in mice with peak neural activity across the acute and persistent phases of post-traumatic headache. Cephalalgia : an international journal of headache, 43(11), 3331024231217469.More infoPost-traumatic headache is very common after a mild traumatic brain injury. Post-traumatic headache may persist for months to years after an injury in a substantial proportion of people. The pathophysiology underlying post-traumatic headache remains unknown but is likely distinct from other headache disorders. Identification of brain areas activated in acute and persistent phases of post-traumatic headache can provide insights into the underlying circuits mediating headache pain. We used an animal model of mild traumatic brain injury-induced post-traumatic headache and c-fos immunohistochemistry to identify brain regions with peak activity levels across the acute and persistent phases of post-traumatic headache.
- Bjorklund, G. R., Anderson, T. R., & Stabenfeldt, S. E. (2021). Recent Advances in Stem Cell Therapies to Address Neuroinflammation, Stem Cell Survival, and the Need for Rehabilitative Therapies to Treat Traumatic Brain Injuries. International journal of molecular sciences, 22(4).More infoTraumatic brain injuries (TBIs) are a significant health problem both in the United States and worldwide with over 27 million cases being reported globally every year. TBIs can vary significantly from a mild TBI with short-term symptoms to a moderate or severe TBI that can result in long-term or life-long detrimental effects. In the case of a moderate to severe TBI, the primary injury causes immediate damage to structural tissue and cellular components. This may be followed by secondary injuries that can be the cause of chronic and debilitating neurodegenerative effects. At present, there are no standard treatments that effectively target the primary or secondary TBI injuries themselves. Current treatment strategies often focus on addressing post-injury symptoms, including the trauma itself as well as the development of cognitive, behavioral, and psychiatric impairment. Additional therapies such as pharmacological, stem cell, and rehabilitative have in some cases shown little to no improvement on their own, but when applied in combination have given encouraging results. In this review, we will abridge and discuss some of the most recent research advances in stem cell therapies, advanced engineered biomaterials used to support stem transplantation, and the role of rehabilitative therapies in TBI treatment. These research examples are intended to form a multi-tiered perspective for stem-cell therapies used to treat TBIs; stem cells and stem cell products to mitigate neuroinflammation and provide neuroprotective effects, biomaterials to support the survival, migration, and integration of transplanted stem cells, and finally rehabilitative therapies to support stem cell integration and compensatory and restorative plasticity.
- Kopruszinski, C. M., Turnes, J. M., Swiokla, J., Weinstein, T. J., Schwedt, T. J., Dodick, D. W., Anderson, T., Navratilova, E., & Porreca, F. (2021). CGRP monoclonal antibody prevents the loss of diffuse noxious inhibitory controls (DNIC) in a mouse model of post-traumatic headache. Cephalalgia : an international journal of headache, 333102420981688.More infoDetermine the role of calcitonin-gene related peptide in promoting post-traumatic headache and dysregulation of central pain modulation induced by mild traumatic brain injury in mice.
- Bharadwaj, V. N., Copeland, C., Mathew, E., Newbern, J., Anderson, T. R., Lifshitz, J., Kodibagkar, V. D., & Stabenfeldt, S. E. (2020). Sex-Dependent Macromolecule and Nanoparticle Delivery in Experimental Brain Injury. Tissue engineering. Part A, 26(13-14), 688-701.More infoThe development of effective therapeutics for brain disorders is challenging, in particular, the blood-brain barrier (BBB) severely limits access of the therapeutics into the brain parenchyma. Traumatic brain injury (TBI) may lead to transient BBB permeability that affords a unique opportunity for therapeutic delivery via intravenous administration ranging from macromolecules to nanoparticles (NPs) for developing precision therapeutics. In this regard, we address critical gaps in understanding the range/size of therapeutics, delivery window(s), and moreover, the potential impact of biological factors for optimal delivery parameters. Here we show, for the first time, to the best of our knowledge, that 24-h postfocal TBI female mice exhibit a heightened macromolecular tracer and NP accumulation compared with male mice, indicating sex-dependent differences in BBB permeability. Furthermore, we report for the first time the potential to deliver NP-based therapeutics within 3 days after focal injury in both female and male mice. The delineation of injury-induced BBB permeability with respect to sex and temporal profile is essential to more accurately tailor time-dependent precision and personalized nanotherapeutics. Impact statement In this study, we identified a sex-dependent temporal profile of blood/brain barrier disruption in a preclinical mouse model of traumatic brain injury (TBI) that contributes to starkly different macromolecule and nanoparticle delivery profiles post-TBI. The implications and potential impact of this work are profound and far reaching as it indicates that a demand of true personalized medicine for TBI is necessary to deliver the right therapeutic at the right time for the right patient.
- Chen, K., Ma, X., Nehme, A., Wei, J., Cui, Y., Cui, Y., Yao, D., Wu, J., Anderson, T., Ferguson, D., Levitt, P., & Qiu, S. (2020). Time-delimited signaling of MET receptor tyrosine kinase regulates cortical circuit development and critical period plasticity. Molecular psychiatry.More infoNormal development of cortical circuits, including experience-dependent cortical maturation and plasticity, requires precise temporal regulation of gene expression and molecular signaling. Such regulation, and the concomitant impact on plasticity and critical periods, is hypothesized to be disrupted in neurodevelopmental disorders. A protein that may serve such a function is the MET receptor tyrosine kinase, which is tightly regulated developmentally in rodents and primates, and exhibits reduced cortical expression in autism spectrum disorder and Rett Syndrome. We found that the peak of MET expression in developing mouse cortex coincides with the heightened period of synaptogenesis, but is precipitously downregulated prior to extensive synapse pruning and certain peak periods of cortical plasticity. These results reflect a potential on-off regulatory synaptic mechanism for specific glutamatergic cortical circuits in which MET is enriched. In order to address the functional significance of the 'off' component of the proposed mechanism, we created a controllable transgenic mouse line that sustains cortical MET signaling. Continued MET expression in cortical excitatory neurons disrupted synaptic protein profiles, altered neuronal morphology, and impaired visual cortex circuit maturation and connectivity. Remarkably, sustained MET signaling eliminates monocular deprivation-induced ocular dominance plasticity during the normal cortical critical period; while ablating MET signaling leads to early closure of critical period plasticity. The results demonstrate a novel mechanism in which temporal regulation of a pleiotropic signaling protein underlies cortical circuit maturation and timing of cortical critical period, features that may be disrupted in neurodevelopmental disorders.
- Holter, M. C., Hewitt, L. T., Nishimura, K. J., Knowles, S. J., Bjorklund, G. R., Shah, S., Fry, N. R., Rees, K. P., Gupta, T. A., Daniels, C. W., Li, G., Marsh, S., Treiman, D. M., Olive, M. F., Anderson, T. R., Sanabria, F., Snider, W. D., & Newbern, J. M. (2021). Hyperactive MEK1 Signaling in Cortical GABAergic Neurons Promotes Embryonic Parvalbumin Neuron Loss and Defects in Behavioral Inhibition. Cerebral cortex (New York, N.Y. : 1991).More infoMany developmental syndromes have been linked to genetic mutations that cause abnormal ERK/MAPK activity; however, the neuropathological effects of hyperactive signaling are not fully understood. Here, we examined whether hyperactivation of MEK1 modifies the development of GABAergic cortical interneurons (CINs), a heterogeneous population of inhibitory neurons necessary for cortical function. We show that GABAergic-neuron specific MEK1 hyperactivation in vivo leads to increased cleaved caspase-3 labeling in a subpopulation of immature neurons in the embryonic subpallial mantle zone. Adult mutants displayed a significant loss of parvalbumin (PV), but not somatostatin, expressing CINs and a reduction in perisomatic inhibitory synapses on excitatory neurons. Surviving mutant PV-CINs maintained a typical fast-spiking phenotype but showed signs of decreased intrinsic excitability that coincided with an increased risk of seizure-like phenotypes. In contrast to other mouse models of PV-CIN loss, we discovered a robust increase in the accumulation of perineuronal nets, an extracellular structure thought to restrict plasticity. Indeed, we found that mutants exhibited a significant impairment in the acquisition of behavioral response inhibition capacity. Overall, our data suggest PV-CIN development is particularly sensitive to hyperactive MEK1 signaling, which may underlie certain neurological deficits frequently observed in ERK/MAPK-linked syndromes.
- Ashina, H., Porreca, F., Anderson, T., Amin, F. M., Ashina, M., Schytz, H. W., & Dodick, D. W. (2019). Post-traumatic headache: epidemiology and pathophysiological insights. Nature reviews. Neurology, 15(10), 607-617.More infoPost-traumatic headache (PTH) is a highly disabling secondary headache disorder and one of the most common sequelae of mild traumatic brain injury, also known as concussion. Considerable overlap exists between PTH and common primary headache disorders. The most common PTH phenotypes are migraine-like headache and tension-type-like headache. A better understanding of the pathophysiological similarities and differences between primary headache disorders and PTH could uncover unique treatment targets for PTH. Although possible underlying mechanisms of PTH have been elucidated, a substantial void remains in our understanding, and further research is needed. In this Review, we describe the evidence from animal and human studies that indicates involvement of several potential mechanisms in the development and persistence of PTH. These mechanisms include impaired descending modulation, neurometabolic changes, neuroinflammation and activation of the trigeminal sensory system. Furthermore, we outline future research directions to establish biomarkers involved in progression from acute to persistent PTH, and we identify potential drug targets to prevent and treat persistent PTH.
- Navratilova, E., Rau, J., Oyarzo, J., Tien, J., Mackenzie, K., Stratton, J., Remeniuk, B., Schwedt, T., Anderson, T., Dodick, D., & Porreca, F. (2019). CGRP-dependent and independent mechanisms of acute and persistent post-traumatic headache following mild traumatic brain injury in mice. Cephalalgia : an international journal of headache, 39(14), 1762-1775.More infoAcute and persistent post-traumatic headache are often debilitating consequences of traumatic brain injury. Underlying physiological mechanisms of post-traumatic headache and its persistence remain unknown, and there are currently no approved therapies for these conditions. Post-traumatic headache often presents with a migraine-like phenotype. As calcitonin-gene related peptide promotes migraine headache, we explored the efficacy and timing of intervention with an anti- calcitonin-gene related peptide monoclonal antibody in novel preclinical models of acute post-traumatic headache and persistent post-traumatic headache following a mild traumatic brain injury event in mice.
- Nichols, J., Perez, R., Wu, C., Adelson, P. D., & Anderson, T. (2015). Traumatic brain injury induces rapid enhancement of cortical excitability in juvenile rats. CNS neuroscience & therapeutics, 21(2), 193-203.More infoFollowing a traumatic brain injury (TBI), 5-50% of patients will develop posttraumatic epilepsy (PTE) with children being particularly susceptible. Currently, PTE cannot be prevented and there is limited understanding of the underlying epileptogenic mechanisms. We hypothesize that early after TBI the brain undergoes distinct cellular and synaptic reorganization that facilitates cortical excitability and promotes the development of epilepsy.