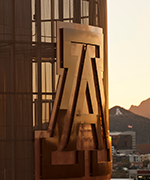
Chad Park
- Associate Professor of Practice
- (520) 626-9388
- Biological Sciences West, Rm. 252
- Tucson, AZ 85721
- ckpark@arizona.edu
Degrees
- Ph.D. Chemical Engineering / Biophysics
- University of California Santa Barbara, Santa Barbara, California, United States
- Study of the Forces and Fusion of Solid and Soft Supported Phospholipid Bilayers
- B.S. Chemical Engineering
- University of Delaware, Newark, Delaware, United States
- Parallelized Monte Carlo Simulation of Square Well Fluids
Awards
- Student Faculty Interaction Award
- University of Arizona, Spring 2019
Interests
Teaching
Student centered learning. Writing for scientists. Molecular structure and visualization for biochemists. TA training.
Research
Lipid structures and signalling related to cell signalling and metabolism. Biophysics. Regulation and variable activity on fiber forming enzymes (not just structural proteins!).
Courses
2024-25 Courses
-
181 Laboratory Preceptor
MCB 391A (Spring 2025) -
Intro Biology I Lab
MCB 181L (Spring 2025) -
181 Laboratory Preceptor
MCB 391A (Fall 2024) -
Intro Biology I Lab
MCB 181L (Fall 2024) -
Special Tutoring Wkshp
MCB 497A (Fall 2024)
2023-24 Courses
-
181 Laboratory Preceptor
MCB 391A (Spring 2024) -
Intro Biology I Lab
MCB 181L (Spring 2024) -
Special Tutoring Wkshp
MCB 497A (Spring 2024) -
181 Laboratory Preceptor
MCB 391A (Fall 2023) -
Internship in Applied Biosci
ABS 593A (Fall 2023) -
Intro Biology I Lab
MCB 181L (Fall 2023) -
Special Tutoring Wkshp
MCB 497A (Fall 2023)
2022-23 Courses
-
181 Laboratory Preceptor
MCB 391A (Spring 2023) -
Internship in Applied Biosci
ABS 593A (Spring 2023) -
Intro Biology I Lab
MCB 181L (Spring 2023) -
Special Tutoring Wkshp
MCB 497A (Spring 2023) -
181 Laboratory Preceptor
MCB 391A (Fall 2022) -
Intro Biology I Lab
MCB 181L (Fall 2022) -
Special Tutoring Wkshp
MCB 497A (Fall 2022)
2021-22 Courses
-
Biochem Lab Techniques
BIOC 463A (Spring 2022) -
Senior Capstone
BIOC 498 (Spring 2022) -
Biochem Lab Techniques
BIOC 463A (Fall 2021) -
Directed Research
BIOC 492 (Fall 2021) -
Honors Preceptorship
BIOC 491H (Fall 2021) -
Visiting Scholars
BIOC 396D (Fall 2021)
2020-21 Courses
-
Biochem Lab Techniques
BIOC 463A (Spring 2021) -
Honors Preceptorship
BIOC 491H (Spring 2021) -
Honors Thesis
BIOC 498H (Spring 2021) -
Preceptorship
BIOC 491 (Spring 2021) -
Senior Capstone
BIOC 498 (Spring 2021) -
Biochem Lab Techniques
BIOC 463A (Fall 2020) -
Honors Preceptorship
BIOC 491H (Fall 2020) -
Honors Thesis
BIOC 498H (Fall 2020) -
Preceptorship
BIOC 491 (Fall 2020) -
Senior Capstone
BIOC 498 (Fall 2020) -
Visiting Scholars
BIOC 396D (Fall 2020)
2019-20 Courses
-
Biochem Lab Techniques
BIOC 463A (Spring 2020) -
Directed Research
BIOC 492 (Spring 2020) -
Honors Preceptorship
BIOC 491H (Spring 2020) -
Preceptorship
BIOC 491 (Spring 2020) -
Biochem Lab Techniques
BIOC 463A (Fall 2019) -
Visiting Scholars
BIOC 396D (Fall 2019)
2018-19 Courses
-
Biochem Lab Techniques
BIOC 463A (Spring 2019) -
Biochem Lab Techniques
BIOC 463A (Fall 2018) -
Visiting Scholars
BIOC 396D (Fall 2018)
2017-18 Courses
-
Biochem Lab Techniques
BIOC 463A (Spring 2018) -
Honors Preceptorship
BIOC 491H (Spring 2018) -
Biochem Lab Techniques
BIOC 463A (Fall 2017) -
Visiting Scholars
BIOC 396D (Fall 2017)
2016-17 Courses
-
Biochem Lab Techniques
BIOC 463A (Spring 2017) -
Biochem Lab Techniques
BIOC 463A (Fall 2016)
2015-16 Courses
-
Fndtns in Biochemistry
BIOC 384 (Summer I 2016) -
Biochemistry
BIOC 462B (Spring 2016)
Scholarly Contributions
Chapters
- Seitz, M., Park, C. K., Wong, J. Y., & Israelachvili, J. N. (1999). Study of the Fusion Process between Solidand Soft-Supported Phospholipid Bilayers with the Surface Forces Apparatus.
Journals/Publications
- Horton, N. C., Marty, M. T., Park, C. K., Prell, J. S., Rolland, A. D., Sanders, H. M., Townsend, J. A., & Wang, J. (2021).
Influenza AM2 Channel Oligomerization Is Sensitive to Its Chemical Environment
. Analytical Chemistry, 93(48), 16273-16281. doi:10.1021/acs.analchem.1c04660More infoViroporins are small viral ion channels that play important roles in the viral infection cycle and are proven antiviral drug targets. Matrix protein 2 from influenza A (AM2) is the best-characterized viroporin, and the current paradigm is that AM2 forms monodisperse tetramers. Here, we used native mass spectrometry and other techniques to characterize the oligomeric state of both the full-length and transmembrane (TM) domain of AM2 in a variety of different pH and detergent conditions. Unexpectedly, we discovered that AM2 formed a range of different oligomeric complexes that were strongly influenced by the local chemical environment. Native mass spectrometry of AM2 in nanodiscs with different lipids showed that lipids also affected the oligomeric states of AM2. Finally, nanodiscs uniquely enabled the measurement of amantadine binding stoichiometries to AM2 in the intact lipid bilayer. These unexpected results reveal that AM2 can form a wider range of oligomeric states than previously thought possible, which may provide new potential mechanisms of influenza pathology and pharmacology. - Horton, N. C., & Park, C. K. (2020).
Novel insights into filament-forming enzymes.
. Nature reviews. Molecular cell biology, 21(1), 1-2. doi:10.1038/s41580-019-0188-1More infoEnzyme filaments are defined as reversible, functional, linear self-assemblies of a single type of enzyme. Filamentation has recently emerged as a new mode of enzymatic regulation. In this Comment, we briefly introduce the diversity and functional consequences of enzyme filamentation. Filamentation is a relatively unknown mode of enzyme regulation, which increases functional diversity. - Horton, N. C., & Park, C. K. (2020).
Novel insights into filament-forming enzymes
. Nature Reviews Molecular Cell Biology, 21(1), 1-2. doi:10.1038/s41580-019-0188-1More infoEnzyme filaments are defined as reversible, functional, linear self-assemblies of a single type of enzyme. Filamentation has recently emerged as a new mode of enzymatic regulation. In this Comment, we briefly introduce the diversity and functional consequences of enzyme filamentation. Filamentation is a relatively unknown mode of enzyme regulation, which increases functional diversity. - Horton, N. C., & Park, C. K. (2019).
Structures, functions, and mechanisms of filament forming enzymes: a renaissance of enzyme filamentation.
. Biophysical reviews, 11(6), 927-994. doi:10.1007/s12551-019-00602-6More infoFilament formation by non-cytoskeletal enzymes has been known for decades, yet only relatively recently has its wide-spread role in enzyme regulation and biology come to be appreciated. This comprehensive review summarizes what is known for each enzyme confirmed to form filamentous structures in vitro, and for the many that are known only to form large self-assemblies within cells. For some enzymes, studies describing both the in vitro filamentous structures and cellular self-assembly formation are also known and described. Special attention is paid to the detailed structures of each type of enzyme filament, as well as the roles the structures play in enzyme regulation and in biology. Where it is known or hypothesized, the advantages conferred by enzyme filamentation are reviewed. Finally, the similarities, differences, and comparison to the SgrAI endonuclease system are also highlighted. - Alcala-Torano, R., Walther, M., Sommer, D. J., Park, C. K., & Ghirlanda, G. (2018). Rational design of a hexameric protein assembly stabilized by metal chelation. Biopolymers, e23233.More infoProtein-based self-assembled nanostructures hold tremendous promise as smart materials. One strategy to control the assembly of individual protein modules takes advantage of the directionality and high affinity bonding afforded by metal chelation. Here, we describe the use of 2,2'-bipyridine units (Bpy) as side chains to template the assembly of large structures (MW approx. 35 000 Da) in a metal-dependent manner. The structures are trimers of independently folded 3-helix bundles, and are held together by 2 Me(Bpy) complexes. The assemblies are stable to thermal denaturation, and are more than 90% helical at 90°C. Circular dichroism spectroscopy shows that one of the 2 possible (Bpy) enantiomers is favored over the other. Because of the sequence pliability of the starting peptides, these constructs could find use to organize functional groups at controlled positions within a supramolecular assembly.
- Alcala‐Torano, R., Alcala‐Torano, R., Ghirlanda, G., Ghirlanda, G., Park, C. K., Park, C. K., Sommer, D. J., Sommer, D. J., Walther, M., & Walther, M. (2018).
Rational design of a hexameric protein assembly stabilized by metal chelation
. Biopolymers, 109(10). doi:10.1002/bip.23233 - Park, C. K., Sanchez, J. L., Barahona, C., Basantes, L. E., Sanchez, J., Hernandez, C., & Horton, N. C. (2018). The run-on oligomer filament enzyme mechanism of SgrAI. Part 1: Assembly kinetics of the run-on oligomer filament. The Journal of biological chemistry.More infoFilament or run-on oligomer formation by metabolic enzymes is now recognized as a widespread phenomenon having potentially unique enzyme regulatory properties and biological roles, and its dysfunction is implicated in human diseases such as cancer, diabetes, and developmental disorders. SgrAI is a bacterial allosteric type II restriction endonuclease that binds to invading phage DNA, may protect the host DNA from off-target cleavage activity, and forms run-on oligomeric filaments with enhanced DNA cleavage activity and altered DNA sequence specificity. However, the mechanisms of SgrAI filament growth, cooperativity in filament formation, sequestration of enzyme activity, and advantages over other filament mechanisms remain unknown. In this first of a two-part series, we developed methods and models to derive association and dissociation rate constants of DNA bound SgrAI in run-on oligomers and addressed the specific questions of cooperativity and filament growth mechanisms. We show that the derived rate constants are consistent with the run-on oligomer sizes determined by EM analysis and are most consistent with a non-cooperative growth mode of the run-on oligomer. These models and methods are extended in Part 2 to include the full DNA cleavage pathway, and address specific questions related to the run-on oligomer mechanism including the sequestration of DNA cleavage activity and trapping of products.
- Park, C. K., Sanchez, J. L., Barahona, C., Basantes, L. E., Sanchez, J., Hernandez, C., & Horton, N. C. (2018). The run-on oligomer filament enzyme mechanism of SgrAI. Part 2: Kinetic modeling of the full DNA cleavage pathway. The Journal of biological chemistry.More infoFilament or run-on oligomer formation by enzymes is now recognized as a widespread phenomenon with potentially unique enzyme regulatory properties and biological roles. SgrAI is an allosteric type II restriction endonuclease that forms run-on oligomeric filaments with activated DNA cleavage activity and altered DNA sequence specificity. In this two-part work, we measure individual steps in the run-on oligomer filament mechanism to address specific questions of cooperativity, trapping, filament growth mechanisms, and sequestration of activity using fluorophore labeled DNA, kinetic FRET measurements, and reaction modeling with global data fitting. The final models and rate constants show that the assembly step involving association of SgrAI/DNA complexes into the run-on oligomer filament is relatively slow (three to four orders of magnitude slower than diffusion limited) and rate limiting at low to moderate concentrations of SgrAI/DNA. The disassembly step involving dissociation of complexes of SgrAI/DNA from each other in the run-on oligomer filament is the next slowest step, but is fast enough to limit the residence time of any one copy of SgrAI or DNA within the dynamic filament. Further, the rate constant for DNA cleavage is found to be four orders of magnitude faster in the run-on oligomer filament than in isolated SgrAI/DNA complexes, and faster than dissociation of SgrAI/DNA complexes from the run-on oligomer filament, making the reaction efficient in that each association into the filament likely leads to DNA cleavage before filament dissociation.
- Shah, S., Dunten, P., Stiteler, A., Park, C. K., & Horton, N. C. (2015). Structure and specificity of FEN-1 from Methanopyrus kandleri. Proteins: Structure, Function, and Bioinformatics, 83(1), 188--194.
- Shah, S., Sanchez, J., Stewart, A., Piperakis, M. M., Cosstick, R., Nichols, C., Park, C. K., Ma, X., Wysocki, V., Bitinaite, J., & others, . (2015). Probing the run-on oligomer of activated SgrAI bound to DNA. PloS one, 10(4), e0124783.
- Zhao, H., Ghirlando, R., Alfonso, C., Arisaka, F., Attali, I., Bain, D. L., Bakhtina, M. M., Becker, D. F., Bedwell, G. J., Bekdemir, A., & others, . (2015). A multilaboratory comparison of calibration accuracy and the performance of external references in analytical ultracentrifugation. PLoS One, 10(5), e0126420.
- Shah, S., Dunten, P., Stiteler, A., Park, C. K., & Horton, N. C. (2014).
Structure and specificity of FEN‐1 from Methanopyrus kandleri
. Proteins: Structure, Function, and Bioinformatics, 83(1), 188-194. doi:10.1002/prot.24704More infoDNA repair is fundamental to genome stability and is found in all three domains of life. However many archaeal species, such as Methanopyrus kandleri, contain only a subset of the eukaryotic nucleotide excision repair (NER) homologs, and those present often contain significant differences compared to their eukaryotic homologs. To clarify the role of the NER XPG-like protein Mk0566 from M. kandleri, its biochemical activity and three-dimensional structure were investigated. Both were found to be more similar to human FEN-1 than human XPG, suggesting a biological role in replication and long-patch base excision repair rather than in NER. Proteins 2015; 83:188–194. © 2014 Wiley Periodicals, Inc. - Bronnimann, M. P., Campos, S. K., Chapman, J. A., & Park, C. K. (2013).
A Transmembrane Domain and GxxxG Motifs within L2 Are Essential for Papillomavirus Infection
. Journal of Virology, 87(1), 464-473. doi:10.1128/jvi.01539-12More infoDuring cellular invasion, human papillomavirus type 16 (HPV16) must transfer its viral genome (vDNA) across the endosomal membrane prior to its accumulation at nuclear PML bodies for the establishment of infection. After cellular uptake, the capsid likely undergoes pH-dependent disassembly within the endo-/lysosomal compartment, thereby exposing hidden domains in L2 that facilitate membrane penetration of L2/vDNA complexes. In an effort to identify regions of L2 that might physically interact with membranes, we have subjected the L2 sequence to multiple transmembrane (TM) domain prediction algorithms. Here, we describe a conserved TM domain within L2 (residues 45 to 67) and investigate its role in HPV16 infection. In vitro, the predicted TM domain adopts an alpha-helical structure in lipid environments and can function as a real TM domain, although not as efficiently as the bona fide TM domain of PDGFR. An L2 double point mutant renders the TM domain nonfunctional and blocks HPV16 infection by preventing endosomal translocation of vDNA. The TM domain contains three highly conserved GxxxG motifs. These motifs can facilitate homotypic and heterotypic interactions between TM helices, activities that may be important for vDNA translocation. Disruption of some of these GxxxG motifs resulted in noninfectious viruses, indicating a critical role in infection. Using a ToxR-based homo-oligomerization assay, we show a propensity for this TM domain to self-associate in a GxxxG-dependent manner. These data suggest an important role for the self-associating L2 TM domain and the conserved GxxxG motifs in the transfer of vDNA across the endo-/lysosomal membrane. - Bronnimann, M. P., Chapman, J. A., Park, C. K., & Campos, S. K. (2013). A transmembrane domain and GxxxG motifs within L2 are essential for papillomavirus infection. Journal of virology, 87(1), 464--473.
- Horton, N. C., Ma, X., Park, C. K., Shah, S., Wysocki, V. H., & Zhou, M. (2013).
Structural Analysis of Activated SgrAI–DNA Oligomers Using Ion Mobility Mass Spectrometry
. Biochemistry, 52(25), 4373-4381. doi:10.1021/bi3013214More infoSgrAI is a type IIF restriction endonuclease that cuts an unusually long recognition sequence and exhibits self-modulation of DNA cleavage activity and sequence specificity. Previous studies have shown that SgrAI forms large oligomers when bound to particular DNA sequences and under the same conditions where SgrAI exhibits accelerated DNA cleavage kinetics. However, the detailed structure and stoichiometry of the SgrAI–DNA complex as well as the basic building block of the oligomers have not been fully characterized. Ion mobility mass spectrometry (IM-MS) was employed to analyze SgrAI–DNA complexes and show that the basic building block of the oligomers is the DNA-bound SgrAI dimer (DBD) with one SgrAI dimer bound to two precleaved duplex DNA molecules each containing one-half of the SgrAI primary recognition sequence. The oligomers contain variable numbers of DBDs with as many as 19 DBDs. Observation of the large oligomers shows that nanoelectrospray ionization (nano-ESI) can preserve the proposed activated form of an enzyme. Finally, the collision cross section of the SgrAI–DNA oligomers measured by IM-MS was found to have a linear relationship with the number of DBDs in each oligomer, suggesting a regular, repeating structure. - Lyumkis, D., Talley, H., Stewart, A. M., Shah, S., Park, C. K., Tama, F., Potter, C. S., Carragher, B., & Horton, N. C. (2013).
Allosteric Regulation of DNA Cleavage and Sequence-Specificity through Run-On Oligomerization
. Structure, 21(10), 1848-1858. doi:10.1016/j.str.2013.08.012More infoSgrAI is a sequence specific DNA endonuclease that functions through an unusual enzymatic mechanism that is allosterically activated 200- to 500-fold by effector DNA, with a concomitant expansion of its DNA sequence specificity. Using single-particle transmission electron microscopy to reconstruct distinct populations of SgrAI oligomers, we show that in the presence of allosteric, activating DNA, the enzyme forms regular, repeating helical structures characterized by the addition of DNA-binding dimeric SgrAI subunits in a run-on manner. We also present the structure of oligomeric SgrAI at 8.6 Å resolution, demonstrating the conformational state of SgrAI in its activated form. Activated and oligomeric SgrAI displays key protein-protein interactions near the helix axis between its N termini, as well as allosteric protein-DNA interactions that are required for enzymatic activation. The hybrid approach reveals an unusual mechanism of enzyme activation that explains SgrAI's oligomerization and allosteric behavior. - Lyumkis, D., Talley, H., Stewart, A., Shah, S., Park, C. K., Tama, F., Potter, C. S., Carragher, B., & Horton, N. C. (2013). Allosteric regulation of DNA cleavage and sequence-specificity through run-on oligomerization. Structure, 21(10), 1848--1858.
- Ma, X., Shah, S., Zhou, M., Park, C. K., Wysocki, V. H., & Horton, N. C. (2013). Structural analysis of activated SgrAI--DNA oligomers using ion mobility mass spectrometry. Biochemistry, 52(25), 4373--4381.
- Briggs, D. B., Giron, R. M., Schnittker, K., Hart, M. V., Park, C. K., Hausrath, A. C., & Tsao, T. (2012). Zinc enhances adiponectin oligomerization to octadecamers but decreases the rate of disulfide bond formation. Biometals, 25(2), 469--486.
- Park, C. K., Stewart, A., Wysocki, V. H., Talley, H., Stewart, A. M., Shah, S., Piperakis, M. M., Park, C. K., Ma, X., Jacovetty, E., Horton, N. C., & Cosstick, R. (2012). Activation by Oligomerization of an Allosteric Sequence Specific Endonuclease. The FASEB Journal, 26.
- Peterson, T. A., Benallie, R. L., Bradford, A. M., Pias, S. C., Yazzie, J., Lor, S. N., Haulsee, Z. M., Park, C. K., Johnson, D. L., Rohrschneider, L. R., & others, . (2012). Dimerization in the Grb7 protein. Journal of Molecular Recognition, 25(8), 427--434.
- Horton, N. C., & Park, C. K. (2010). Crystallization of zinc finger proteins bound to DNA. Engineered Zinc Finger Proteins: Methods and Protocols, 457--477.
- Park, C. K., Joshi, H. K., Agrawal, A., Ghare, M. I., Little, E. J., Dunten, P. W., Bitinaite, J., & Horton, N. C. (2010). Domain swapping in allosteric modulation of DNA specificity. PLoS biology, 8(12), e1000554.
- Park, C. K., Stiteler, A. P., Shah, S., Ghare, M. I., Bitinaite, J., & Horton, N. C. (2010). Activation of DNA Cleavage by Oligomerization of DNA-Bound SgrAI. Biochemistry, 49(41), 8818-8830. doi:10.1021/bi100557v
- Park, C. K., Stiteler, A. P., Shah, S., Ghare, M. I., Bitinaite, J., & Horton, N. C. (2010). Activation of DNA cleavage by oligomerization of DNA-bound SgrAI. Biochemistry, 49(41), 8818--8830.
- Georgieva, T., Devanathan, S., Stropova, D., Park, C. K., Salamon, Z., Tollin, G., Hruby, V. J., Roeske, W. R., Yamamura, H. I., & Varga, E. (2008). Unique agonist-bound cannabinoid CB 1 receptor conformations indicate agonist specificity in signaling. European journal of pharmacology, 581(1), 19--29.
- Lee, Y. S., Petrov, R., Park, C. K., Ma, S., Davis, P., Lai, J., Porreca, F., Vardanyan, R., & Hruby, V. J. (2007). Development of novel enkephalin analogues that have enhanced opioid activities at both $mu$ and $delta$ opioid receptors. Journal of medicinal chemistry, 50(22), 5528--5532.
- Alves, I. D., Park, C. K., & Hruby, V. J. (2005). Plasmon resonance methods in GPCR signaling and other membrane events. Current Protein and Peptide Science, 6(4), 293--312.
- Alcantar, N. A., Park, C., Pan, J., & Israelachvili, J. N. (2003). Adhesion and coalescence of ductile metal surfaces and nanoparticles. Acta materialia, 51(1), 31--47.
- Seitz, M., Park, C. K., Wong, J. Y., & Israelachvili, J. N. (2001). Long-range interaction forces between polymer-supported lipid bilayer membranes. Langmuir, 17(15), 4616--4626.
- Seitz, M., Ter-Ovanesyan, E., Hausch, M., Park, C. K., Zasadzinski, J. A., Zentel, R., & Israelachvili, J. N. (2000). Formation of tethered supported bilayers by vesicle fusion onto lipopolymer monolayers promoted by osmotic stress. Langmuir, 16(14), 6067--6070.
- Park, C. K., Schmitt, F. J., Evert, L., Schwartz, D. K., Israelachvili, J. N., & Knobler, C. M. (1999). Film balance and fluorescence microscopic investigation of the effects of Ca2+ on mixed DMPC/DMPG monolayers. Langmuir, 15(1), 202--206.
- Wong, J. Y., Majewski, J., Seitz, M., Park, C. K., Israelachvili, J. N., & Smith, G. S. (1999). Polymer-cushioned bilayers. I. A structural study of various preparation methods using neutron reflectometry. Biophysical Journal, 77(3), 1445--1457.
- Wong, J. Y., Park, C. K., Seitz, M., & Israelachvili, J. (1999). Polymer-cushioned bilayers. II. An investigation of interaction forces and fusion using the surface forces apparatus. Biophysical journal, 77(3), 1458--1468.
- Majewski, J., Wong, J. Y., Park, C. K., Seitz, M., Israelachvili, J. N., & Smith, G. S. (1998). Structural studies of polymer-cushioned lipid bilayers. Biophysical Journal, 75(5), 2363--2367.
- Schmitt, F., Park, C., Simon, J., Ringsdorf, H., & Israelachvili, J. (1998). Direct surface force and contact angle measurements of an adsorbed polymer with a lower critical solution temperature. Langmuir, 14(10), 2838--2845.
- Seitz, M., Wong, J. Y., Park, C. K., Alcantar, N. A., & Israelachvili, J. (1998). Formation of tethered supported bilayers via membrane-inserting reactive lipids. Thin solid films, 327, 767--771.
- Campbell, S. E., Park, C. K., Lasic, D. D., & Israelachvili, J. N. (1997). Structure and Forces in Transfection Related Surfactant Systems. MRS Online Proceedings Library Archive, 489.