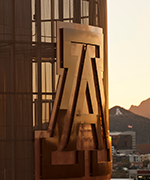
Konrad E Zinsmaier
- Department Head, Neuroscience
- Professor, Neuroscience
- Professor, Molecular and Cellular Biology
- Professor, Entomology / Insect Science - GIDP
- Professor, Physiological Sciences - GIDP
- Member of the Graduate Faculty
- Professor, Neuroscience - GIDP
- (520) 626-1343
- Gould-Simpson, Rm. 627
- Tucson, AZ 85721
- kez4@arizona.edu
Biography
My laboratory has a long-standing interest in understanding molecular mechanisms that facilitate synaptic function and plasticity, and mechanisms that protect synapses from neurodegeneration. In addition, we are interested in the specialized mechanisms of mitochondrial biology in axons and dendrites of neurons. We investigate these fundamental mechanisms by undertaking a multidisciplinary approach, exploiting the neuromuscular junction (NMJ) of genetically modified Drosophila as a model system. Currently, our research focuses on a) neuroprotective mechanism of synapses, b) molecular mechanisms that control regulated neurotransmitter release, and c) molecular mechanisms of mitochondrial transport and biology in axons. These projects use forward and reverse genetics to genetically dissect the role of key components that are conserved from invertebrates to mammals. Abnormal function is assayed by a variety of techniques including electrical recordings, electron microscopy, confocal microscopy, live imaging of intracellular calcium, endo- and exocytosis, and mitochondrial transport in axons of motor neurons.
Degrees
- Dr. rer. nat. Neuroscience and Genetics
- Julius Maximilian Universität, Würzburg, Bavaria, Germany
- Klonierung und Charakterisierung des immunhistochemisch identifizierten csp Gens von Drosophila melanogaster
Work Experience
- University of Arizona, Tucson, Arizona (2009 - Ongoing)
- University of Arizona, Tucson, Arizona (2008 - Ongoing)
- University of Arizona, Tucson, Arizona (2008 - Ongoing)
- University of Arizona, Tucson, Arizona (2008 - 2009)
- University of Arizona, Tucson, Arizona (2002 - 2008)
- University of Arizona, Tucson, Arizona (2002 - 2008)
- University of Pennsylvania School of Medicine (1995 - 2002)
- California Institute of Technology, Pasadena, California (1993 - 1995)
- California Institute of Technology, Pasadena, California (1990 - 1993)
Interests
Teaching
aspects of molecular and cellular neuroscienceaspects of neurogeneticsmolecular mechanisms underlying neurological and neurodegenerative diseases
Research
- neuroprotective mechanisms- molecular mechanisms of synaptic function and plasticity- molecular mechanisms of mitochondrial transport and survival in axons and dendrites of neurons
Courses
2025-26 Courses
-
Molecular Mech Learning/Memory
NROS 412 (Fall 2025) -
Neurogenetics
NROS 430 (Fall 2025) -
Preceptorship
NROS 491 (Fall 2025)
2024-25 Courses
-
Directed Research
NROS 392 (Spring 2025) -
Directed Research
NROS 492 (Spring 2025) -
Honors Directed Research
NROS 492H (Spring 2025) -
Honors Internship
NROS 493H (Spring 2025) -
Honors Preceptorship
NROS 491H (Spring 2025) -
Honors Thesis
NROS 498H (Spring 2025) -
Independent Study
NROS 299 (Spring 2025) -
Internship
NROS 393 (Spring 2025) -
Internship
NROS 493 (Spring 2025) -
Neurogenetics
NROS 430 (Spring 2025) -
Neuroscience Colloquium
NRSC 695F (Spring 2025) -
Neuroscience Colloquium
NRSC 695G (Spring 2025) -
Preceptorship
NROS 491 (Spring 2025) -
Directed Research
NROS 392 (Fall 2024) -
Directed Research
NROS 492 (Fall 2024) -
Honors Directed Research
NROS 392H (Fall 2024) -
Honors Directed Research
NROS 492H (Fall 2024) -
Honors Internship
NROS 393H (Fall 2024) -
Honors Thesis
NROS 498H (Fall 2024) -
Independent Study
NROS 299 (Fall 2024) -
Independent Study
NROS 399 (Fall 2024) -
Internship
NROS 393 (Fall 2024) -
Molecular Mech Learning/Memory
NROS 412 (Fall 2024) -
Neurogenetics
NROS 430 (Fall 2024) -
Prin Cell+Molec Neurobio
MCB 588 (Fall 2024) -
Prin Cell+Molec Neurobio
NRSC 588 (Fall 2024)
2023-24 Courses
-
Honors Directed Research
NROS 392H (Spring 2024) -
Honors Preceptorship
NROS 491H (Spring 2024) -
Independent Study
NROS 199 (Spring 2024) -
Independent Study
NROS 299 (Spring 2024) -
Independent Study
NROS 399 (Spring 2024) -
Internship
NROS 493 (Spring 2024) -
Neurogenetics
NROS 430 (Spring 2024) -
Preceptorship
NROS 491 (Spring 2024) -
Senior Capstone
BIOC 498 (Spring 2024) -
Directed Research
ECOL 492 (Fall 2023) -
Directed Research
NROS 492 (Fall 2023) -
Honors Independent Study
NROS 399H (Fall 2023) -
Independent Study
NROS 499 (Fall 2023) -
Molecular Mech Learning/Memory
NROS 412 (Fall 2023) -
Neurogenetics
NROS 430 (Fall 2023) -
Senior Capstone
BIOC 498 (Fall 2023)
2022-23 Courses
-
Directed Research
NSCS 492 (Spring 2023) -
Independent Study
MCB 399 (Spring 2023) -
Neurogenetics
NROS 430 (Spring 2023) -
Preceptorship
NROS 491 (Spring 2023) -
Independent Study
NROS 399 (Fall 2022) -
Prin Cell+Molec Neurobio
BIOC 588 (Fall 2022) -
Prin Cell+Molec Neurobio
NRSC 588 (Fall 2022) -
Prin Cell+Molec Neurobio
PSIO 588 (Fall 2022)
2021-22 Courses
-
Directed Research
NSCS 392 (Spring 2022) -
Honors Independent Study
MCB 399H (Spring 2022) -
Honors Thesis
NSCS 498H (Spring 2022) -
Neurogenetics
NROS 430 (Spring 2022) -
Directed Rsrch
MCB 392 (Fall 2021) -
Honors Thesis
NSCS 498H (Fall 2021) -
Neuroscience Colloquium
NRSC 695F (Fall 2021) -
Neuroscience Colloquium
NRSC 695G (Fall 2021) -
Prin Cell+Molec Neurobio
CMM 588 (Fall 2021) -
Prin Cell+Molec Neurobio
EIS 588 (Fall 2021) -
Prin Cell+Molec Neurobio
MCB 588 (Fall 2021) -
Prin Cell+Molec Neurobio
NRSC 588 (Fall 2021) -
Prin Cell+Molec Neurobio
PSIO 588 (Fall 2021)
2020-21 Courses
-
Directed Research
NSCS 392 (Spring 2021) -
Directed Research
NSCS 492 (Spring 2021) -
Honors Independent Study
NSCS 399H (Spring 2021) -
Independent Study
NSCS 299 (Spring 2021) -
Neurogenetics
NROS 430 (Spring 2021) -
Neuroscience Colloquium
NRSC 695F (Spring 2021) -
Neuroscience Colloquium
NRSC 695G (Spring 2021) -
Directed Research
NSCS 392 (Fall 2020) -
Directed Research
NSCS 492 (Fall 2020) -
Engaging Topics in NSCS
NSCS 195B (Fall 2020) -
Independent Study
NSCS 299 (Fall 2020) -
Neuroscience Colloquium
NRSC 695F (Fall 2020) -
Neuroscience Colloquium
NRSC 695G (Fall 2020) -
Prin Cell+Molec Neurobio
BIOC 588 (Fall 2020) -
Prin Cell+Molec Neurobio
CMM 588 (Fall 2020) -
Prin Cell+Molec Neurobio
MCB 588 (Fall 2020) -
Prin Cell+Molec Neurobio
NRSC 588 (Fall 2020)
2019-20 Courses
-
Directed Research
NSCS 392 (Spring 2020) -
Directed Research
PSIO 492 (Spring 2020) -
Honors Independent Study
NSCS 399H (Spring 2020) -
Neurogenetics
NROS 430 (Spring 2020) -
Neuroscience Colloquium
NRSC 695F (Spring 2020) -
Neuroscience Colloquium
NRSC 695G (Spring 2020) -
Directed Research
NSCS 392 (Fall 2019) -
Engaging Topics in NSCS
NSCS 195B (Fall 2019) -
Honors Independent Study
NSCS 399H (Fall 2019) -
Independent Study
NSCS 399 (Fall 2019) -
Neuroscience Colloquium
NRSC 695F (Fall 2019) -
Neuroscience Colloquium
NRSC 695G (Fall 2019) -
Prin Cell+Molec Neurobio
CMM 588 (Fall 2019) -
Prin Cell+Molec Neurobio
EIS 588 (Fall 2019) -
Prin Cell+Molec Neurobio
MCB 588 (Fall 2019) -
Prin Cell+Molec Neurobio
NRSC 588 (Fall 2019)
2018-19 Courses
-
Directed Research
NSCS 392 (Summer I 2019) -
Directed Research
NSCS 392 (Spring 2019) -
Dissertation
NRSC 920 (Spring 2019) -
Honors Independent Study
NSCS 399H (Spring 2019) -
Independent Study
NSCS 399 (Spring 2019) -
Neurogenetics
NROS 430 (Spring 2019) -
Neuroscience Colloquium
NRSC 695F (Spring 2019) -
Neuroscience Colloquium
NRSC 695G (Spring 2019) -
Dissertation
NRSC 920 (Fall 2018) -
Honors Independent Study
NSCS 399H (Fall 2018) -
Neuroscience Colloquium
NRSC 695F (Fall 2018) -
Neuroscience Colloquium
NRSC 695G (Fall 2018) -
Prin Cell+Molec Neurobio
BIOC 588 (Fall 2018) -
Prin Cell+Molec Neurobio
MCB 588 (Fall 2018) -
Prin Cell+Molec Neurobio
NRSC 588 (Fall 2018) -
Prin Cell+Molec Neurobio
PSIO 588 (Fall 2018)
2017-18 Courses
-
Directed Research
BIOC 492 (Spring 2018) -
Directed Rsrch
MCB 392 (Spring 2018) -
Dissertation
NRSC 920 (Spring 2018) -
Honors Independent Study
MCB 499H (Spring 2018) -
Honors Thesis
NSCS 498H (Spring 2018) -
Independent Study
ECOL 499 (Spring 2018) -
Independent Study
NSCS 299 (Spring 2018) -
Independent Study
NSCS 399 (Spring 2018) -
Neurogenetics
NROS 430 (Spring 2018) -
Neuroscience Colloquium
NRSC 695F (Spring 2018) -
Neuroscience Colloquium
NRSC 695G (Spring 2018) -
Thesis
MCB 910 (Spring 2018) -
Directed Research
BIOC 492 (Fall 2017) -
Directed Rsrch
MCB 492 (Fall 2017) -
Dissertation
NRSC 920 (Fall 2017) -
Honors Independent Study
NSCS 499H (Fall 2017) -
Honors Thesis
NSCS 498H (Fall 2017) -
Independent Study
ECOL 499 (Fall 2017) -
Independent Study
NSCS 399 (Fall 2017) -
Introduction to Research
MCB 795A (Fall 2017) -
Neuroscience Colloquium
NRSC 695F (Fall 2017) -
Neuroscience Colloquium
NRSC 695G (Fall 2017) -
Prin Cell+Molec Neurobio
CMM 588 (Fall 2017) -
Prin Cell+Molec Neurobio
EIS 588 (Fall 2017) -
Prin Cell+Molec Neurobio
MCB 588 (Fall 2017) -
Prin Cell+Molec Neurobio
NRSC 588 (Fall 2017) -
Research
MCB 900 (Fall 2017) -
Thesis
MCB 910 (Fall 2017)
2016-17 Courses
-
Directed Rsrch
MCB 392 (Summer I 2017) -
Dissertation
NRSC 920 (Spring 2017) -
Honors Thesis
NSCS 498H (Spring 2017) -
Honors Thesis
PSIO 498H (Spring 2017) -
Methods In Neuroscience
NRSC 700 (Spring 2017) -
Neurogenetics
NROS 430 (Spring 2017) -
Neuroscience Colloquium
NRSC 695F (Spring 2017) -
Neuroscience Colloquium
NRSC 695G (Spring 2017) -
Thesis
MCB 910 (Spring 2017) -
Directed Research
NROS 492 (Fall 2016) -
Directed Research
NSCS 392 (Fall 2016) -
Directed Research
NSCS 492 (Fall 2016) -
Dissertation
NRSC 920 (Fall 2016) -
Honors Independent Study
PSIO 499H (Fall 2016) -
Honors Thesis
NSCS 498H (Fall 2016) -
Honors Thesis
PSIO 498H (Fall 2016) -
Neuroscience Colloquium
NRSC 695F (Fall 2016) -
Neuroscience Colloquium
NRSC 695G (Fall 2016) -
Prin Cell+Molec Neurobio
BIOC 588 (Fall 2016) -
Prin Cell+Molec Neurobio
MCB 588 (Fall 2016) -
Prin Cell+Molec Neurobio
NRSC 588 (Fall 2016)
2015-16 Courses
-
Dissertation
NRSC 920 (Summer I 2016) -
Directed Research
NSCS 492 (Spring 2016) -
Dissertation
NRSC 920 (Spring 2016) -
Honors Independent Study
NSCS 299H (Spring 2016) -
Honors Thesis
MCB 498H (Spring 2016) -
Honors Thesis
NSCS 498H (Spring 2016) -
Neurogenetics
NROS 430 (Spring 2016) -
Neuroscience Colloquium
NRSC 695F (Spring 2016) -
Neuroscience Colloquium
NRSC 695G (Spring 2016)
Scholarly Contributions
Chapters
- Zinsmaier, K. E. (2011). Cysteine-string protein’s role at the synapse. In Folding for the synapse(pp 145-176). New York: Springer.
- Zinsmaier, K. E. (1997). Cysteine-String Proteins.. In Guidebook to Molecular Chaperones and Protein Folding Sequences(pp 115-117). Oxford: Oxford University Press.
- Zinsmaier, K. E. (1991). A novel EF-hand Ca2+ -binding protein is expressed in discrete subsets of muscle and nerve cells of Drosophila.. In Novel Calcium-Binding Proteins(pp 505-510). Berlin: Springer-Verlag.
Journals/Publications
- Zinsmaier, K. E., Ye, Y., Xu, M., Saidi, L. F., Xu, Y., & Lee, J. (2022). Abnormal triaging of misfolded proteins by adult neuronal ceroid lipofuscinosis-associated DNAJC5/CSPα mutants causes lipofuscin accumulation. Autophagy, 19(1), 204-223. doi:10.1080/15548627.2022.2065618More infoMutations in DNAJC5/CSPα are associated with adult neuronal ceroid lipofuscinosis (ANCL), a dominant-inherited neurodegenerative disease featuring lysosome-derived autofluorescent storage materials (AFSMs) termed lipofuscin. Functionally, DNAJC5 has been implicated in chaperoning synaptic proteins and in misfolding-associated protein secretion (MAPS), but how DNAJC5 dysfunction causes lipofuscinosis and neurodegeneration is unclear. Here we report two functionally distinct but coupled chaperoning activities of DNAJC5, which jointly regulate lysosomal homeostasis: While endolysosome-associated DNAJC5 promotes ESCRT-dependent microautophagy, a fraction of perinuclear and non-lysosomal DNAJC5 mediates MAPS. Functional proteomics identifies a previously unknown DNAJC5 interactor SLC3A2/CD98hc that is essential for the perinuclear DNAJC5 localization and MAPS but dispensable for microautophagy. Importantly, uncoupling these two processes, as seen in cells lacking SLC3A2 or expressing ANCL-associated DNAJC5 mutants, generates DNAJC5-containing AFSMs resembling NCL patient-derived lipofuscin and induces neurodegeneration in a Drosophila ANCL model. These findings suggest that MAPS safeguards microautophagy to avoid DNAJC5-associated lipofuscinosis and neurodegeneration.Abbreviations: 3-MA: 3-methyladenine; ACTB: actin beta; AFSM: autofluorescent storage materials; ANCL: adult neuronal ceroid lipofuscinosis; Baf. A1: bafilomycin A1; CLN: ceroid lipofuscinosis neuronal; CLU: clusterin; CS: cysteine string domain of DNAJC5/CSPα; CUPS: compartment for unconventional protein secretion; DN: dominant negative; DNAJC5/CSPα: DnaJ heat shock protein family (Hsp40) member C5; eMI: endosomal microautophagy; ESCRT: endosomal sorting complex required for transport; GFP: green fluorescent protein; HSPA8/HSC70: heat shock protein family A (Hsp70) member 8; INCL: infant neuronal ceroid lipofuscinosis; JNCL: juvenile neuronal ceroid lipofuscinosis; KO: knockout; LAMP1: lysosomal associated membrane protein 1; LAPTM4B: lysosomal protein transmembrane 4 beta; LN: linker domain of DNAJC5/CSPα; MAPS: misfolding-associated protein secretion; mCh/Ch: mCherry; mCi/Ci: mCitrine; MTOR: mechanistic target of rapamycin kinase; NCL: neuronal ceroid lipofuscinosis; PPT1: palmitoyl-protein thioesterase 1; PQC: protein quality control; SBP: streptavidin binding protein; SGT: small glutamine-rich tetratricopeptide repeat; shRNA: short hairpin RNA; SLC3A2/CD98hc: solute carrier family 3 member 2; SNCA/α-synuclein: synuclein alpha; TMED10: transmembrane p24 trafficking protein 10; UV: ultraviolet; VPS4: vacuolar protein sorting 4 homolog; WT: wild type.
- Zinsmaier, K. E., Ye, Y., Xu, M., Saidi, L. F., Xu, Y., & Lee, J. (2022). Abnormal triaging of misfolded proteins by adult neuronal ceroid lipofuscinosis-associated DNAJC5/CSPα mutants causes lipofuscin accumulation. Autophagy, 19(1), 204-223. doi:10.1080/15548627.2022.2065618More infoMutations in DNAJC5/CSPα are associated with adult neuronal ceroid lipofuscinosis (ANCL), a dominant-inherited neurodegenerative disease featuring lysosome-derived autofluorescent storage materials (AFSMs) termed lipofuscin. Functionally, DNAJC5 has been implicated in chaperoning synaptic proteins and in misfolding-associated protein secretion (MAPS), but how DNAJC5 dysfunction causes lipofuscinosis and neurodegeneration is unclear. Here we report two functionally distinct but coupled chaperoning activities of DNAJC5, which jointly regulate lysosomal homeostasis: While endolysosome-associated DNAJC5 promotes ESCRT-dependent microautophagy, a fraction of perinuclear and non-lysosomal DNAJC5 mediates MAPS. Functional proteomics identifies a previously unknown DNAJC5 interactor SLC3A2/CD98hc that is essential for the perinuclear DNAJC5 localization and MAPS but dispensable for microautophagy. Importantly, uncoupling these two processes, as seen in cells lacking SLC3A2 or expressing ANCL-associated DNAJC5 mutants, generates DNAJC5-containing AFSMs resembling NCL patient-derived lipofuscin and induces neurodegeneration in a Drosophila ANCL model. These findings suggest that MAPS safeguards microautophagy to avoid DNAJC5-associated lipofuscinosis and neurodegeneration.Abbreviations: 3-MA: 3-methyladenine; ACTB: actin beta; AFSM: autofluorescent storage materials; ANCL: adult neuronal ceroid lipofuscinosis; Baf. A1: bafilomycin A1; CLN: ceroid lipofuscinosis neuronal; CLU: clusterin; CS: cysteine string domain of DNAJC5/CSPα; CUPS: compartment for unconventional protein secretion; DN: dominant negative; DNAJC5/CSPα: DnaJ heat shock protein family (Hsp40) member C5; eMI: endosomal microautophagy; ESCRT: endosomal sorting complex required for transport; GFP: green fluorescent protein; HSPA8/HSC70: heat shock protein family A (Hsp70) member 8; INCL: infant neuronal ceroid lipofuscinosis; JNCL: juvenile neuronal ceroid lipofuscinosis; KO: knockout; LAMP1: lysosomal associated membrane protein 1; LAPTM4B: lysosomal protein transmembrane 4 beta; LN: linker domain of DNAJC5/CSPα; MAPS: misfolding-associated protein secretion; mCh/Ch: mCherry; mCi/Ci: mCitrine; MTOR: mechanistic target of rapamycin kinase; NCL: neuronal ceroid lipofuscinosis; PPT1: palmitoyl-protein thioesterase 1; PQC: protein quality control; SBP: streptavidin binding protein; SGT: small glutamine-rich tetratricopeptide repeat; shRNA: short hairpin RNA; SLC3A2/CD98hc: solute carrier family 3 member 2; SNCA/α-synuclein: synuclein alpha; TMED10: transmembrane p24 trafficking protein 10; UV: ultraviolet; VPS4: vacuolar protein sorting 4 homolog; WT: wild type.
- Zinsmaier, K. E. (2020). Mitochondrial Miro GTPases coordinate mitochondrial and peroxisomal dynamics.. Small GTPases, 1-27. doi:10.1080/21541248.2020.1843957More infoMitochondria and peroxisomes are highly dynamic, multifunctional organelles. Both perform key roles for cellular physiology and homoeostasis by mediating bioenergetics, biosynthesis, and/or signalling. To support cellular function, they must be properly distributed, of proper size, and be able to interact with other organelles. Accumulating evidence suggests that the small atypical GTPase Miro provides a central signalling node to coordinate mitochondrial as well as peroxisomal dynamics. In this review, I summarize our current understanding of Miro-dependent functions and molecular mechanisms underlying the proper distribution, size and function of mitochondria and peroxisomes.
- Imler, E., Torvund, M., Zinsmaier, K. E., Chandra, S. S., Zhang, Y., Kindelay, S., & Pyon, J. S. (2019).
A Drosophila model of neuronal ceroid lipofuscinosis CLN4 reveals a hypermorphic gain of function mechanism
. eLife, 8. doi:10.7554/elife.46607More infoThe autosomal dominant neuronal ceroid lipofuscinoses (NCL) CLN4 is caused by mutations in the synaptic vesicle (SV) protein CSPα. We developed animal models of CLN4 by expressing CLN4 mutant human CSPα (hCSPα) in Drosophila neurons. Similar to patients, CLN4 mutations induced excessive oligomerization of hCSPα and premature lethality in a dose-dependent manner. Instead of being localized to SVs, most CLN4 mutant hCSPα accumulated abnormally, and co-localized with ubiquitinated proteins and the prelysosomal markers HRS and LAMP1. Ultrastructural examination revealed frequent abnormal membrane structures in axons and neuronal somata. The lethality, oligomerization and prelysosomal accumulation induced by CLN4 mutations was attenuated by reducing endogenous wild type (WT) dCSP levels and enhanced by increasing WT levels. Furthermore, reducing the gene dosage of Hsc70 also attenuated CLN4 phenotypes. Taken together, we suggest that CLN4 alleles resemble dominant hypermorphic gain of function mutations that drive excessive oligomerization and impair membrane trafficking. - Imler, E., Zhang, Y., Zinsmaier, K. E., Zhang, Y. Q., Torvund, M., Pyon, J. S., Kindelay, S., Imler, E., & Chandra, S. S. (2019). A Drosophila model of neuronal ceroid lipofuscinosis CLN4 reveals a hypermorphic gain of function mechanism.. eLife, 8. doi:10.7554/elife.46607More infoThe autosomal dominant neuronal ceroid lipofuscinoses (NCL) CLN4 is caused by mutations in the synaptic vesicle (SV) protein CSPα. We developed animal models of CLN4 by expressing CLN4 mutant human CSPα (hCSPα) in Drosophila neurons. Similar to patients, CLN4 mutations induced excessive oligomerization of hCSPα and premature lethality in a dose-dependent manner. Instead of being localized to SVs, most CLN4 mutant hCSPα accumulated abnormally, and co-localized with ubiquitinated proteins and the prelysosomal markers HRS and LAMP1. Ultrastructural examination revealed frequent abnormal membrane structures in axons and neuronal somata. The lethality, oligomerization and prelysosomal accumulation induced by CLN4 mutations was attenuated by reducing endogenous wild type (WT) dCSP levels and enhanced by increasing WT levels. Furthermore, reducing the gene dosage of Hsc70 also attenuated CLN4 phenotypes. Taken together, we suggest that CLN4 alleles resemble dominant hypermorphic gain of function mutations that drive excessive oligomerization and impair membrane trafficking.
- Zinsmaier, K. E., Young, K., Torvund, M., Rogers, G. C., Patron, L. A., Nagatomo, K., Imad, M., Guo, X., & Eves, D. T. (2019). Cul4 ubiquitin ligase cofactor DCAF12 promotes neurotransmitter release and homeostatic plasticity.. The Journal of Cell Biology, 218(3), 993-1010. doi:10.1083/jcb.201805099More infoWe genetically characterized the synaptic role of the Drosophila homologue of human DCAF12, a putative cofactor of Cullin4 (Cul4) ubiquitin ligase complexes. Deletion of Drosophila DCAF12 impairs larval locomotion and arrests development. At larval neuromuscular junctions (NMJs), DCAF12 is expressed presynaptically in synaptic boutons, axons, and nuclei of motor neurons. Postsynaptically, DCAF12 is expressed in muscle nuclei and facilitates Cul4-dependent ubiquitination. Genetic experiments identified several mechanistically independent functions of DCAF12 at larval NMJs. First, presynaptic DCAF12 promotes evoked neurotransmitter release. Second, postsynaptic DCAF12 negatively controls the synaptic levels of the glutamate receptor subunits GluRIIA, GluRIIC, and GluRIID. The down-regulation of synaptic GluRIIA subunits by nuclear DCAF12 requires Cul4. Third, presynaptic DCAF12 is required for the expression of synaptic homeostatic potentiation. We suggest that DCAF12 and Cul4 are critical for normal synaptic function and plasticity at larval NMJs.
- Coyne, A., Lorenzini, I., Chou, C., Torvund, M., Rogers, R., Starr, A., Zaepfel, B., Johannesmeyer, J., Nishimune, H., Zinsmaier, K. E., Rossoll, W., Sattler, R., & Zarnescu, D. C. (2017). Post-transcriptional inhibition of Hsc70-4/HSPA8 expression leads to synaptic vesicle cycling defects in multiple models of ALS. Cell Reports.More infoAmyotrophic Lateral Sclerosis (ALS) is a synaptopathy accompanied by the presence of cytoplasmic aggregates containing TDP-43, an RNA binding protein linked to ~97% of ALS cases. Using a Drosophila model of ALS, we show that TDP-43 overexpression in motor neurons results in decreased expression of the Hsc70-4 chaperone at the neuromuscular junction (NMJ). Mechanistically, mutant TDP-43 sequesters hsc70-4 mRNA and impairs its translation. Expression of Hsc70-4’s ortholog, HSPA8 is also reduced in primary motor neurons and NMJs of mice expressing mutant TDP-43. Electrophysiology, imaging, and genetic interaction experiments reveal TDP-43 dependent defects in synaptic vesicle endocytosis. These deficits can be partially restored by overexpression of Hsc70-4, cysteine-string protein (Csp) or dynamin. This suggests that TDP-43 toxicity results in part from impaired activity of the synaptic CSP/Hsc70 chaperone complex impacting dynamin function. Finally, Hsc70-4/HSPA8 expression is also post-transcriptionally reduced in fly and human iPSC C9ORF72 models, suggesting a common disease pathomechanism.
- Patrón, L. A., & Zinsmaier, K. E. (2016). Mitochondria on the Road to Power Axonal Regeneration. Neuron, 92(6), 1152-1154.More infoIn this issue of Neuron, Han et al. (2016) and Cartoni et al. (2016) define a critical role of mitochondrial transport for successful axon regeneration after injury and provide new insights into intrinsic mechanisms controlling neuronal regeneration capacity in worms and mice.
- Babic, M., Russo, G. J., Andrea, W., Sangston, R., Gonzales, M., & Zinsmaier, K. E. (2015). Miro's N-terminal GTPase domain is required for transport of mitochondria into axons and dendrites. J. Neurosci. doi:10.1523/JNEUROSCI.1035-14.2015
- Zhou, Y., Wong, C., Cho, K., van der Hoeven, D., Liang, H., Thakur, D. P., Luo, J., Babic, M., Zinsmaier, K. E., Zhu, M. X., Hu, H., Venkatachalam, K., & Hancock, J. F. (2015). Membrane potential modulates plasma membrane phospholipid dynamics and K-Ras signaling. Science, 349(6250), 873-6. doi:1126/science.aaa5619More infoPlasma membrane depolarization can trigger cell proliferation, but how membrane potential influences mitogenic signaling is uncertain. Here, we show that plasma membrane depolarization induces nanoscale reorganization of phosphatidylserine and phosphatidylinositol 4,5-bisphosphate but not other anionic phospholipids. K-Ras, which is targeted to the plasma membrane by electrostatic interactions with phosphatidylserine, in turn undergoes enhanced nanoclustering. Depolarization-induced changes in phosphatidylserine and K-Ras plasma membrane organization occur in fibroblasts, excitable neuroblastoma cells, and Drosophila neurons in vivo and robustly amplify K-Ras-dependent mitogen-activated protein kinase (MAPK) signaling. Conversely, plasma membrane repolarization disrupts K-Ras nanoclustering and inhibits MAPK signaling. By responding to voltage-induced changes in phosphatidylserine spatiotemporal dynamics, K-Ras nanoclusters set up the plasma membrane as a biological field-effect transistor, allowing membrane potential to control the gain in mitogenic signaling circuits.
- Imler, E., & Zinsmaier, K. E. (2014). TRPV1 channels: not so inactive on the ER. Neuron, 84(4), 659-61.More infoIn this issue of Neuron, Wong et al. (2014) report a remarkable evolutionarily conserved role for the Drosophila TRPV1 homolog Inactive controlling synaptic growth at larval neuromuscular junctions by facilitating Ca(2+) release from the endoplasmic reticulum.
- Liu, Y., Pearce, M. W., Honda, T., Johnson, T. K., Charlu, S., Sharma, K. R., Imad, M., Burke, R. E., Zinsmaier, K. E., Ray, A., Dahanukar, A., Bruyne, M. d., & Warr, C. G. (2014). The Drosophila melanogaster Phospholipid Flippase dATP8B Is Required for Odorant Receptor Function. PLoS Genetics, 10(3).More infoAbstract: The olfactory systems of insects are fundamental to all aspects of their behaviour, and insect olfactory receptor neurons (ORNs) exhibit exquisite specificity and sensitivity to a wide range of environmental cues. In Drosophila melanogaster, ORN responses are determined by three different receptor families, the odorant (Or), ionotropic-like (IR) and gustatory (Gr) receptors. However, the precise mechanisms of signalling by these different receptor families are not fully understood. Here we report the unexpected finding that the type 4 P-type ATPase phospholipid transporter dATP8B, the homologue of a protein associated with intrahepatic cholestasis and hearing loss in humans, is crucial for Drosophila olfactory responses. Mutations in dATP8B severely attenuate sensitivity of odorant detection specifically in Or-expressing ORNs, but do not affect responses mediated by IR or Gr receptors. Accordingly, we find dATP8B to be expressed in ORNs and localised to the dendritic membrane of the olfactory neurons where signal transduction occurs. Localisation of Or proteins to the dendrites is unaffected in dATP8B mutants, as is dendrite morphology, suggesting instead that dATP8B is critical for Or signalling. As dATP8B is a member of the phospholipid flippase family of ATPases, which function to determine asymmetry in phospholipid composition between the outer and inner leaflets of plasma membranes, our findings suggest a requirement for phospholipid asymmetry in the signalling of a specific family of chemoreceptor proteins. © 2014 Liu et al.
- Tsai, P., Course, M. M., Lovas, J. R., Hsieh, C., Babic, M., Zinsmaier, K. E., & Wang, X. (2014). PINK1-mediated phosphorylation of Miro inhibits synaptic growth and protects dopaminergic neurons in Drosophila. Scientific reports, 4, 6962.More infoMutations in the mitochondrial Ser/Thr kinase PINK1 cause Parkinson's disease. One of the substrates of PINK1 is the outer mitochondrial membrane protein Miro, which regulates mitochondrial transport. In this study, we uncovered novel physiological functions of PINK1-mediated phosphorylation of Miro, using Drosophila as a model. We replaced endogenous Drosophila Miro (DMiro) with transgenically expressed wildtype, or mutant DMiro predicted to resist PINK1-mediated phosphorylation. We found that the expression of phospho-resistant DMiro in a DMiro null mutant background phenocopied a subset of phenotypes of PINK1 null. Specifically, phospho-resistant DMiro increased mitochondrial movement and synaptic growth at larval neuromuscular junctions, and decreased the number of dopaminergic neurons in adult brains. Therefore, PINK1 may inhibit synaptic growth and protect dopaminergic neurons by phosphorylating DMiro. Furthermore, muscle degeneration, swollen mitochondria and locomotor defects found in PINK1 null flies were not observed in phospho-resistant DMiro flies. Thus, our study established an in vivo platform to define functional consequences of PINK1-mediated phosphorylation of its substrates.
- Babic, M., & Zinsmaier, K. E. (2011). Memory, synapse stability, and β-adducin. Neuron, 69(6), 1039-1041.More infoPMID: 21435549;Abstract: In this issue of Neuron, two studies by Pielage et al. and Bednarek and Caroni suggest that the cytoskeleton regulator β-Adducin provides an activity-dependent switch controlling synapse disassembly and assembly at the Drosophila neuromuscular junction (NMJ) and the mouse hippocampus. In mice, the β-Adducin switch is required for the improvement of learning and memory induced by enriched environments. © 2011 Elsevier Inc.
- Zinsmaier, K. E. (2011). Drosophila Neurobiology: A Laboratory Manual. The Quarterly Review of Biology, 86, 351-352.
- Chouhan, A. K., Zhang, J., Zinsmaier, K. E., & Macleod, G. T. (2010). Presynaptic mitochondria in functionally different motor neurons exhibit similar affinities for Ca2+ uptake but exert little influence as Ca2+ buffers at nerve firing rates in situ. Journal of Neuroscience, 30(5), 1869-1881.More infoPMID: 20130196;PMCID: PMC2824909;Abstract: Mitochondria accumulate within nerve terminals and support synaptic function, most notably through ATP production. They can also sequester Ca 2+ during nerve stimulation, but it is unknown whether this limits presynaptic Ca2+ levels at physiological nerve firing rates. Similarly, it is unclear whether mitochondrial Ca2+ sequestration differs between functionally different nerve terminals. We addressed these questions using a combination of synthetic and genetically encoded Ca 2+ indicators to examine cytosolic and mitochondrial Ca2+ levels in presynaptic terminals of tonic (MN13-Ib) and phasic (MNSNb/d-Is) motor neurons in Drosophila, which, as we determined, fire during fictive locomotion at ∼42 Hz and ∼8 Hz, respectively. Mitochondrial Ca2+ sequestration starts in both terminals at ∼250 nM, exhibits a similar Ca2+-uptake affinity (∼410 nM), and does not require Ca 2+ release from the endoplasmic reticulum. Nonetheless, mitochondrial Ca2+ uptake in type Is terminals is more responsive to low-frequency nerve stimulation and this is due to higher cytosolic Ca2+ levels. Since type Ib terminals have a higher mitochondrial density than Is terminals, it seemed possible that greater mitochondrial Ca2+ sequestration may be responsible for the lower cytosolic Ca2+ levels in Ib terminals. However, genetic and pharmacological manipulations of mitochondrial Ca 2+ uptake did not significantly alter nerve-stimulated elevations in cytosolic Ca2+ levels in either terminal type within physiologically relevant rates of stimulation. Our findings indicate that presynaptic mitochondria have a similar affinity for Ca2+ in functionally different nerve terminals, but do not limit cytosolic Ca2+ levels within the range of motor neuron firing rates in situ. Copyright © 2010 the authors.
- Zinsmaier, K. E. (2010). Cysteine-string protein's neuroprotective role. Journal of Neurogenetics, 24(3), 120-132.More infoPMID: 20583963;Abstract: Cysteine-string protein (CSP), a member of the DnaJ/Hsp40 family of cochaperones, is critical for maintaining neurotransmitter release and preventing neurodegeneration. CSP likely forms a chaperone complex on synaptic vesicles together with the 70-kDa heat shock cognate (Hsc70) and the small glutamine-rich tetratricopeptide repeat (TPR)-containing protein (SGT) that may control or protect the assembly and activity of SNARE proteins and various other protein substrates. Here, the author summarizes studies that elucidated CSP's neuroprotective role. © 2010 Informa Healthcare USA, Inc.
- Cziko, A. J., McCann, C. T., Howlett, I. C., Barbee, S. A., Duncan, R. P., Luedemann, R., Zarnescu, D., Zinsmaier, K. E., Parker, R. R., & Ramaswami, M. (2009). Genetic modifiers of dFMR1 encode RNA granule components in Drosophila. Genetics, 182(4), 1051-1060.More infoPMID: 19487564;PMCID: PMC2728847;Abstract: Mechanisms of neuronal mRNA localization and translation are of considerable biological interest. Spatially regulated mRNA translation contributes to cell-fate decisions and axon guidance during development, as well as to long-term synaptic plasticity in adulthood. The Fragile-X Mental Retardation protein (FMRP/dFMR1) is one of the best-studied neuronal translational control molecules and here we describe the identification and early characterization of proteins likely to function in the dFMR1 pathway. Induction of the dFMR1 in sevenless-expressing cells of the Drosophila eye causes a disorganized (rough) eye through a mechanism that requires residues necessary for dFMR1/FMRP's translational repressor function. Several mutations in dco, orb2, pAbp, rm62, and smD3 genes dominantly suppress the sev-dfmr1 rough-eye phenotype, suggesting that they are required for dFMR1-mediated processes. The encoded proteins localize to dFMR1-containing neuronal mRNPs in neurites of cultured neurons, and/or have an effect on dendritic branching predicted for bona fide neuronal translational repressors. Genetic mosaic analyses indicate that dco, orb2, rm62, smD3, and dfmr1 are dispensable for translational repression of hid, a microRNA target gene, known to be repressed in wing discs by the bantam miRNA. Thus, the encoded proteins may function as miRNA- and/or mRNA-specific translational regulators in vivo. Copyright © 2009 by the Genetics Society of America.
- Russo, G. J., Louie, K., Wellington, A., Macleod, G. T., Fangle, H. u., Panchumarthi, S., & Zinsmaier, K. E. (2009). Drosophila Miro is required for both anterograde and retrograde axonal mitochondrial transport. Journal of Neuroscience, 29(17), 5443-5455.More infoPMID: 19403812;PMCID: PMC2693725;Abstract: Microtubule-based transport of mitochondria into dendrites and axons is vital for sustaining neuronal function. Transport along microtubule tracks proceeds in a series of plus and minus end-directed movements that are facilitated by kinesin and dynein motors. How the opposing movements are controlled to achieve effective transport over large distances remains unclear. Previous studies showed that the conserved mitochondrial GTPase Miro is required for mitochondrial transport into axons and dendrites and serves as a Ca 2+ sensor that controls mitochondrial mobility. To directly examine Miro's significance for kinesin- and/or dynein-mediated mitochondrial motility, we live-imaged movements of GFP-tagged mitochondria in larval Drosophila motor axons upon genetic manipulations of Miro. Loss of Drosophila Miro (dMiro) reduced the effectiveness of both anterograde and retrograde mitochondrial transport by selectively impairing kinesin- or dynein-mediated movements, depending on the direction of net transport. Net anterogradely transported mitochondria exhibited reduced kinesin- but normal dynein-mediated movements. Net retrogradely transported mitochondria exhibitedmuchshorter dynein-mediated movements, whereas kinesin-mediated movements were minimally affected. In both cases, the duration of short stationary phases increased proportionally. Overexpression (OE) of dMiro also impaired the effectiveness of mitochondrial transport. Finally, loss and OE of dMiro altered the length of mitochondria in axons through a mechanistically separate pathway. We suggest that dMiro promotes effective antero- and retrograde mitochondrial transport by extending the processivity of kinesin and dynein motors according to a mitochondrion's programmed direction of transport. Copyright © 2009 Society for Neuroscience.
- Zinsmaier, K. E., Babic, M., & Russo, G. J. (2009). Mitochondrial transport dynamics in axons and dendrites. Results and Problems in Cell Differentiation, 48, 107-139.More infoPMID: 19582407;Abstract: Mitochondrial dynamics and transport have emerged as key factors in the regulation of neuronal differentiation and survival. Mitochondria are dynamically transported in and out of axons and dendrites to maintain neuronal and synaptic function. Transport proceeds through a controlled series of plus- and minus-end directed movements along microtubule tracks (MTs) that are often interrupted by short stops. This bidirectional motility of mitochondria is facilitated by plus end-directed kinesin and minus end-directed dynein motors, and may be coordinated and controlled by a number of mechanisms that integrate intracellular signals to ensure efficient transport and targeting of mitochondria. In this chapter, we discuss our understanding of mechanisms that facilitate mitochondrial transport and delivery to specific target sites in dendrites and axons. © 2009 Springer-Verlag Berlin Heidelberg.
- Zinsmaier, K., Zinsmaier, K. E., & Zarnescu, D. C. (2009). Ferrying wingless across the synaptic cleft. Cell, 139(2).More infoSecreted Wnt morphogens mediate cell-cell communication, but the mechanism of Wnt transfer between cells is unknown. Korkut et al. (2009) report that the transmembrane protein Evi is a versatile carrier that guides Wingless to presynaptic terminals of motor neurons and then escorts it across the synaptic cleft. In postsynaptic muscles, Evi promotes Frizzled-2 trafficking.
- Louie, K., Russo, G. J., Salkoff, D. B., Wellington, A., & Zinsmaier, K. E. (2008). Effects of imaging conditions on mitochondrial transport and length in larval motor axons of Drosophila. Comparative Biochemistry and Physiology - A Molecular and Integrative Physiology, 151(2), 159-172.More infoPMID: 18639643;PMCID: PMC2607117;Abstract: The distribution of mitochondria is sensitive to physiological stresses and changes in metabolic demands. Consequently, it is important to carefully define the conditions facilitating live imaging of mitochondrial transport in dissected animal preparations. In this study, we examined Schneider's and the haemolymph-like solutions HL3 and HL6 for their suitability to image mitochondrial transport in motor axons of dissected Drosophila melanogaster larvae. Overall, mitochondrial transport kinetics in larval motor axons appeared similar among all three solutions. Unexpectedly, HL3 solution selectively increased the length of mitochondria in the context of the net-direction of transport. We also found that mitochondrial transport is sensitive to the extracellular Ca2+ but not glutamate concentration. High concentrations of extracellular glutamate affected only the ratio between motile and stationary mitochondria. Our study offers a valuable overview of mitochondrial transport kinetics in larval motor axons of Drosophila under various conditions, guiding future studies genetically dissecting mechanisms of mitochondrial transport. © 2008 Elsevier Inc. All rights reserved.
- Dawson-Scully, K., Lin, Y., Imad, M., Zhang, J., Marin, L., Horne, J. A., Meinertzhagen, I. A., Karunanithi, S., Zinsmaier, K. E., & Atwood, H. L. (2007). Morphological and functional effects of altered cysteine string protein at the Drosophila larval neuromuscular junction. Synapse, 61(1), 1-16.More infoPMID: 17068777;Abstract: The synaptic vesicle-associated cysteine string protein (CSP) is critical for neurotransmitter release at the neuromuscular junction (NMJ) of Drosophila, where the ∼4% of mutant flies lacking CSP that survive to adulthood exhibit spastic jumping and shaking, temperature-sensitive paralysis, and premature death. Previously, it has been shown that CSP is also required for nerve terminal growth and the prevention of neurodegeneration in Drosophila and mice. At larval csp null mutant NMJs of Drosophila, intracellular recordings from the muscle showed that evoked release is significantly reduced at room temperature. However, it remained unclear whether the reduction in evoked release might be due to a loss of synaptic boutons, loss of synapses, and alterations in trafficking of vesicles to synapses. To resolve these issues, we have examined synaptic structure and function of csp null mutant NMJs at the level of single boutons, csp null mutations proportionally reduce the number of synaptic boutons of both motor neurons (1s and 1b) innervating larval muscles 6 and 7, while the number of synapses per bouton remains normal. However, focal recordings from individual synaptic boutons show that nerve-evoked neurotransmitter release is also impaired in both 1s and 1b boutons. Further, our ultrastructural analyses show that the reduction in evoked release at low stimulation frequencies is not due to a loss of synapses or to alterations in docked vesicles at synapses. Together, these data suggest that CSP promotes synaptic growth and evoked neurotransmitter release by mechanistically independent signaling pathways. © 2006 Wiley-Liss, Inc.
- Macleod, G. T., & Zinsmaier, K. E. (2006). Synaptic Homeostasis on the Fast Track. Neuron, 52(4), 569-571.More infoPMID: 17114040;Abstract: Synaptic homeostasis is a phenomenon that prevents the nervous system from descending into chaos. In this issue of Neuron, Frank et al. overturn the notion that synaptic homeostasis at Drosophila NMJs is a slow developmental process. They report that postsynaptic changes are offset within minutes by a homeostatic increase in neurotransmitter release that requires the presynaptic Ca2+ channel Cacophony. © 2006 Elsevier Inc. All rights reserved.
- Zinsmaier, K. E. (2006). ELLIOT S. VALENSTEIN. The War of the Soups and Sparks: The Discovery of Neurotransmitters and the Dispute over How Nerves Communicate. New York, Columbia University Press, 2005. 256 pp. (No price given).. Journal of the History of Medicine and Allied Sciences. doi:10.1093/jhmas/jrj050More infoValenstein’s The War of the Soups and Sparks portrays an exceptionally polarizing debate that separated the emerging field of neuroscience into two camps: neurophysiologists and pharmacologists. The matter of their dispute was one of neuroscience’s most important questions: how do neurons communicate with one another? As we understand it today, the nervous system forms neural circuits by connecting individual neurons through a vast array of highly specialized cell-cell contact sites—called synapses—that use two different modes of operation. Electrical synapses have the obvious advantage of transmitting signals without substantial delay, while the degree of modulating signal strength is rather limited. In contrast, chemical signal transmission is delayed by the secretion of neurotransmitters, but nevertheless provides a better means of computing the encoded information. Valenstein’s book describes the long and remarkably controversial development of this understanding. Around 1900, it became generally accepted that neurons are connected by synapses that leave a physical gap between cells. Initially, almost all neurophysiologists believed that the main mode of signal transmission in the brain had to be electrical (sparks). The concept of chemical transmission was developed in stages, and its general acceptance in the scientific community was marked by the old mistake of unreasonable opposition to new ideas. The first hint came from the observation that acetylcholine and adrenalin affect the function of organs in a way similar to the stimulation of the innervating nerves; the famous example is the slower heart rate produced by stimulating the vagus nerve. Although this was firmly established by Henry Dale’s work, the idea that the innervating nerve might secrete chemicals was not seriously considered until Otto Loewi’s discovery of the “Vagusstoff” in 1921, which led to the concept of neurotransmitters (soups). Since Loewi’s work could not identify the origin of the secreted Vagusstoff, and because of the difficulties encountered in repeating his experiment, his work initially encountered vehement skepticism. Using a better system, Dale identified the Vagusstoff as acetylcholine and showed that it was indeed released from the nerve. However, despite the awarding of the Nobel Prize to Loewi and Dell in 1936, chemical transmission was still considered to be an isolated case. Neurophysiologists, like John Eccles, disputed the idea that transmitters might also mediate rapid signal transmission in the brain. For a number of years, the debate was fought vigorously but respectfully. A breakthrough occurred when Eccles, one of the staunchest opponents of chemical transmission, provided key evidence that synaptic transmission between motor nerves and skeletal muscles is indeed chemical, admitting that he and many others had been wrong all along. Ironically, just when the idea blossomed that all synapses might use neurotransmitters, a few electrical synapses were finally identified in the brain. In the end, less than one percent of all synapses turned out to rely on “sparks.”
- Zinsmaier, K. E. (2006). Elliot S. Valenstein. The War of the Soups and Sparks: The Discovery of Neurotransmitters and the Dispute over How Nerves Communicate. J Hist Med Allied Sci, 61, 408-410.More infoValenstein’s The War of the Soups and Sparks portrays an exceptionally polarizing debate that separated the emerging field of neuroscience into two camps: neurophysiologists and pharmacologists. The matter of their dispute was one of neuroscience’s most important questions: how do neurons communicate with one another?
- Bronk, P., Nie, Z., Klose, M. K., Dawson-Scully, K., Zhang, J., Robertson, R. M., Atwood, H. L., & Zinsmaier, K. E. (2005). The multiple functions of cysteine-string protein analyzed at Drosophila nerve terminals. Journal of Neuroscience, 25(9), 2204-2214.More infoPMID: 15745946;Abstract: The synaptic vesicle-associated cysteine-string protein (CSP) is important for synaptic transmission. Previous studies revealed multiple defects at neuromuscular junctions (NMJs) of csp null-mutant Drosophila, but whether these defects are independent of each other or mechanistically linked through J domain mediated-interactions with heat-shock cognate protein 70 (Hsc70) has not been established. To resolve this issue, we genetically dissected the individual functions of CSP by an in vivo structure/function analysis. Expression of mutant CSP lacking the J domain at csp null-mutant NMJs fully restored normal thermo-tolerance of evoked transmitter release but did not completely restore evoked release at room temperature and failed to reverse the abnormal intraterminal Ca2+ levels. This suggests that J domain-mediated functions are essential for the regulation of intraterminal Ca2+ levels but only partially required for regulating evoked release and not required for protecting evoked release against thermal stress. Hence, CSP can also act as an Hsc70-independent chaperone protecting evoked release from thermal stress. Expression of mutant CSP lacking the L domain restored neurotransmission and partially reversed the abnormal intraterminal Ca 2+ levels, suggesting that the L domain is important, although not essential, for the role of CSP in regulating intraterminal Ca2+ levels. We detected no effects of csp mutations on individual presynaptic Ca2+ signals triggered by action potentials, suggesting that presynaptic Ca2+ entry is not primarily impaired. Both the J and L domains were also required for the role of CSP in synaptic growth. Together, these results suggest that CSP has several independent synaptic functions, affecting synaptic growth, evoked release, thermal protection of evoked release, and intraterminal Ca2+ levels at rest and during stimulation. Copyright © 2005 Society for Neuroscience.
- Zinsmaier, K., Zinsmaier, K. E., Guo, X., Macleod, G. T., Wellington, A., Hu, F., Panchumarthi, S., Schoenfield, M., Marin, L., Charlton, M. P., & Atwood, H. L. (2005). The GTPase dMiro is required for axonal transport of mitochondria to Drosophila synapses. Neuron, 47(3).More infoWe have identified EMS-induced mutations in Drosophila Miro (dMiro), an atypical mitochondrial GTPase that is orthologous to human Miro (hMiro). Mutant dmiro animals exhibit defects in locomotion and die prematurely. Mitochondria in dmiro mutant muscles and neurons are abnormally distributed. Instead of being transported into axons and dendrites, mitochondria accumulate in parallel rows in neuronal somata. Mutant neuromuscular junctions (NMJs) lack presynaptic mitochondria, but neurotransmitter release and acute Ca2+ buffering is only impaired during prolonged stimulation. Neuronal, but not muscular, expression of dMiro in dmiro mutants restored viability, transport of mitochondria to NMJs, the structure of synaptic boutons, the organization of presynaptic microtubules, and the size of postsynaptic muscles. In addition, gain of dMiro function causes an abnormal accumulation of mitochondria in distal synaptic boutons of NMJs. Together, our findings suggest that dMiro is required for controlling anterograde transport of mitochondria and their proper distribution within nerve terminals.
- Song, W., & Zinsmaier, K. E. (2003). Endophilin and synaptojanin hook up to promote synaptic vesicle endocytosis. Neuron, 40(4), 665-667.More infoPMID: 14622570;Abstract: Clathrin-mediated endocytosis of synaptic vesicles requires molecular rearrangements of proteins as well as lipids. In this issue of Neuron, Schuske et al. and Verstreken et al. show that the lipid-modifying enzyme endophilin recruits and stabilizes the polyphosphoinositide phosphatase synaptojanin at nerve terminals. This remarkable pairing of two enzymatic activities promotes multiple steps of clathrin-mediated endocytosis of synaptic vesicles.
- Song, W., Ranjan, R., Dawson-Scully, K., Bronk, P., Marin, L., Seroude, L., Lin, Y., Nie, Z., Atwood, H. L., Benzer, S., & Zinsmaier, K. E. (2002). Presynaptic regulation of neurotransmission in Drosophila by the G protein-coupled receptor Methuselah. Neuron, 36(1), 105-119.More infoPMID: 12367510;Abstract: Regulation of synaptic strength is essential for neuronal information processing, but the molecular mechanisms that control changes in neuroexocytosis are only partially known. Here we show that the putative G protein-coupled receptor Methuselah (Mth) is required in the presynaptic motor neuron to acutely upregulate neurotransmitter exocytosis at larval Drosophila NMJs. Mutations in the mth gene reduce evoked neurotransmitter release by ∼50%, and decrease synaptic area and the density of docked and clustered vesicles. Pre- but not postsynaptic expression of normal Mth restored normal release in mth mutants. Conditional expression of Mth restored normal release and normal vesicle docking and clustering but not the reduced size of synaptic sites, suggesting that Mth acutely adjusts vesicle trafficking to synaptic sites.
- Bronk, P., Wenniger, J. J., Dawson-Scully, K., Guo, X., Hong, S., Atwood, H. L., & Zinsmaier, K. E. (2001). Drosophila Hsc70-4 is critical for neurotransmitter exocytosis in vivo. Neuron, 30(2), 475-488.More infoPMID: 11395008;Abstract: Previous in vitro studies of cysteine-string protein (CSP) imply a potential role for the clathrin-uncoating ATPase Hsc70 in exocytosis. We show that hypomorphic mutations in Drosophila Hsc70-4 (Hsc4) impair nerve-evoked neurotransmitter release, but not synaptic vesicle recycling in vivo. The loss of release can be restored by increasing external or internal Ca2+ and is caused by a reduced Ca2+ sensitivity of exocytosis downstream of Ca2+ entry. Hsc4 and CSP are likely to act in common pathways, as indicated by their in vitro protein interaction, the similar loss of evoked release in individual and double mutants, and genetic interactions causing a loss of release in trans-heterozygous hsc4-csp double mutants. We suggest that Hsc4 and CSP cooperatively augment the probability of release by increasing the Ca2+ sensitivity of vesicle fusion.
- Zinsmaier, K. E., & Bronk, P. (2001). Molecular chaperones and the regulation of neurotransmitter exocytosis. Biochemical Pharmacology, 62(1), 1-11.More infoPMID: 11377391;Abstract: Regulated neurotransmitter release depends on a precise sequence of events that lead to repeated cycles of exocytosis and endocytosis. These events are mediated by a series of molecular interactions among vesicular, plasma membrane, and cytosolic proteins. An emerging theme has been that molecular chaperones may guide the sequential restructuring of stable or transient protein complexes to promote a temporal and spatial regulation of the endo- and exocytotic machinery and to ensure a vectorial passage through the vesicle cycle. Chaperones, specialized for a few substrates, are ideally suited to participate in regulatory processes that require some molecular dexterity to rearrange conformational or oligomeric protein structures. This article emphasizes the significance of three molecular chaperone systems in regulated neurotransmitter release: the regulation of soluble NSF attachment protein receptor (SNARE) complexes by N-ethylmaleimide-sensitive factor (NSF) and the soluble NSF attachment protein (SNAP), the uncoating of clathrin-coated vesicles by the 70 kDa heat-shock cognate protein (Hsc70), and the regulation of SNARE complex-associated protein interactions by cysteine-string protein and Hsc70. © 2001 Elsevier Science Inc.
- Dawson-Scully, K., Bronk, P., Atwood, H. L., & Zinsmaier, K. E. (2000). Cysteine-string protein increases the calcium sensitivity of neurotransmitter exocytosis in Drosophila. Journal of Neuroscience, 20(16), 6039-6047.More infoPMID: 10934253;Abstract: Previous studies suggest that the vesicular s-string protein (CSP) may modulate presynaptic Ca2+ channel activity in fast neurotransmitter release. To test this hypothesis, we analyzed the dynamics of presynaptic Ca2+ ion influx with the Ca2+ indicator fluo-4 AM at csp mutant neuromuscular junctions of Drosophila. From 24 to 30°C, stimulus-evoked, relative presynaptic Ca2+ signals were increasingly larger in csp mutant boutons than in controls. Above 30°C, Ca2+ signals declined and were similar to controls at 34°C. A prolonged decay of Ca2+ signals in mutant boutons at high temperatures indicated abnormally slow Ca2+ clearance. Cytosolic Ca2+ at rest was determined with the ratiometric Ca2+ indicator fura-2 AM and was similar in mutant and control boutons at 24°C but higher in mutant boutons at 34°C. Despite larger Ca2+ signals in mutant boutons, evoked neurotransmitter release was always reduced in csp mutants and exhibited pronounced facilitation. Thus, a lack of Ca2+ entry cannot explain the reduction of neurotransmitter release in csp mutants. At all temperatures tested, raising extracellular Ca2+ increased transmitter release elicited by single stimuli in csp mutants. Collectively, these data suggest multiple functions for CSP at synaptic terminals. Increased Ca2+ signals coupled with reduced release suggest a direct function of CSP in exocytosis downstream from Ca2+ entry. Because the reduction of evoked release in csp mutants is counteracted by increased Ca2+ levels, we suggest that CSP primarily increases the Ca2+ sensitivity of the exocytotic machinery.
- Nie, Z., Ranjan, R., Wenniger, J. J., Hong, S. N., Bronk, P., & Zinsmaier, K. E. (1999). Overexpression of cysteine-string proteins in Drosophila reveals interactions with syntaxin. Journal of Neuroscience, 19(23), 10270-10279.More infoPMID: 10575024;Abstract: Cysteine-string proteins (CSPs) are associated with secretory vesicles and critical for regulated neurotransmitter release and peptide exocytosis. At nerve terminals, CSPs have been implicated in the mediation of neurotransmitter exocytosis by modulating presynaptic calcium channels; however, studies of CSPs in peptidergic secretion suggest a direct role in exocytosis independent of calcium transmembrane fluxes. Here we show that the individual expression of various CSP isoforms in Drosophila similarly rescues the loss of evoked neurotransmitter release at csp null mutant motor nerve terminals, suggesting widely overlapping functions for each isoform. Thus, the structural difference of CSP variants may not explain the opposing putative functions of CSP in neurotransmitter and peptide exocytosis. Consistently, the individual overexpression of each CSP isoform in wild-type Drosophila shows similar effects such as impaired viability and interference with wing and eye development. The dominant effects caused by the overexpression of CSP are suppressed by the simultaneous overexpression of syntaxin-lA but not by the coexpression of SNAP-25. Although overexpression of CSP itself has no apparent effect on the synaptic physiology of larval motor nerve terminals, it fully suppresses the decrease of evoked release induced by the overexpression of syntaxin-1A. A direct protein-protein interaction of CSP with syntaxin is further supported by coimmunoprecipitations of syntaxin with CSP and by protein binding assays using recombinant fusion proteins. Together, the genetic and biochemical interactions of CSP and syntaxin-1A suggest that CSP may chaperone or modulate protein-protein interactions of syntaxin-1A with either calcium channels or other components of the regulatory machinery mediating depolarization-dependent neurotransmitter exocytosis.
- Eberle, K. K., Zinsmaier, K. E., Buchner, S., Gruhn, M., Jenni, M., Arnold, C., Leibold, C., Reisch, D., Walter, N., Hafen, E., Hofbauer, A., Pflugfelder, G. O., & Buchner, E. (1998). Wide distribution of the cysteine string proteins in Drosophila tissues revealed by targeted mutagenesis. Cell and Tissue Research, 294(2), 203-217.More infoPMID: 9799436;Abstract: The 'cysteine string protein' (CSP) genes of higher eukaryotes code for a novel family of proteins characterized by a 'J' domain and an unusual cysteine-rich region. Previous studies had localized the proteins in neuropil and synaptic terminals of larval and adult Drosophila and linked the temperature-sensitive paralysis of the mutants described here to conditional failure of synaptic transmission. We now use the null mutants as negative controls in order to reliably detect even low concentrations of CSPs by immunohistochemistry, employing three monoclonal antibodies. In wild-type flies high levels of cysteine string proteins are found not only in apparently all synaptic terminals of the embryonic, larval, and adult nervous systems, but also in the 'tall cells' of the cardia, in the follicle cells of the ovary, in specific structures of the female spermatheca, and in the male testis and ejaculatory bulb. In addition, low levels of CSPs appear to be present in all tissues examined, including neuronal perikarya, axons, muscles, Malpighian tubules, and salivary glands. Western blots of isolated tissues demonstrate that of the four isoforms expressed in heads only the largest is found in non-neural organs. The wide expression of CSPs suggests that at least some of the various phenotypes of the null mutants observed at permissive temperatures, such as delayed development, short adult lifespan, modified electroretinogram, and optomotor behavior, may be caused by the lack of CSPs outside synaptic terminals.
- Ranjan, R., Bronk, P., & Zinsmaier, K. E. (1998). Cysteine string protein is required for calcium secretion coupling of evoked neurotransmission in Drosophila but not for vesicle recycling. Journal of Neuroscience, 18(3), 956-964.More infoPMID: 9437017;Abstract: The entire deletion of the cysteine string protein (CSP) gene causes a temperature-sensitive (ts) block of evoked neurotransmission in Drosophila. CSP has been found to interact in vitro with the clathrin-uncoating ATPase HSC70, suggesting a potential role of CSP in vesicle recycling. Using FM1-43 imaging, we analyzed whether the ts block of neurotransmission in csp mutants is caused by a defect in vesicle exocytosis or vesicle recycling. We determined that FM1-43-labeled synaptic boutons of csp mutant neuromuscular junctions fail to destain at 32°C after K+ depolarization, and that FM1-43 dye uptake cannot be evoked by K+ stimulation at 32°C. However, when we stimulated dye uptake independent of depolarization by using black widow spider venom (BWSV), we observed endocytotic uptake of FM1-43. This suggests that endocytosis exhibits no primary ts defect. In addition, we found no ts defect of vesicle recycling at 32°C that would correlate with the ts block of neurotransmission. We also discovered that BWSV and the calcium ionophore calcimycin stimulate FM1-43 destaining and quantal release in csp mutants at 32°C when depolarization fails to evoke any response. The wild-type-like, calcimycin-induced response in csp null mutants indicates that some aspect of the depolarization-dependent calcium signaling pathway must be impaired, either calcium entry, calcium action, or both. Collectively, our results indicate that the csp mutation affects calcium secretion coupling of evoked exocytosis but not vesicle recycling. This supports the hypothesis that CSP links synaptic vesicles to calcium secretion coupling.
- Umbach, J. A., Zinsmaier, K. E., Eberle, K. K., Buchner, E., Benzer, S., & Gundersen, C. B. (1994). Presynaptic dysfunction in Drosophila csp mutants. Neuron, 13(4), 899-907.More infoPMID: 7946336;Abstract: Cysteine string proteins are synapse-specific proteins. In Drosophila, csp deletion mutants exhibit temperature-sensitive paralysis and early death. Here, we report that neuromuscular transmission is impaired presynaptically in these csp mutant larvae. At 22°C, evoked transmitter release is depressed relative to wild type and rescued controls, and high frequency stimulation of the nerve leads to sporadic failures. At 30°C, stimulus-evoked responses decline gradually before failing completely. When the temperature is returned to 22°C, evoked responses recover. Spontaneous release events persist at both 22°C and 30°C. Since nerve conduction and postsynaptic sensitivity are unaffected, these data indicate that csp mutations disrupt depolarization- secretion coupling. This disruption explains the cellular basis of the temperature-sensitive paralysis of these organisms.
- Zinsmaier, K. E., Eberle, K. K., Buchner, E., Walter, N., & Benzer, S. (1994). Paralysis and early death in cysteine string protein mutants of Drosophila. Science, 263(5149), 977-980.More infoPMID: 8310297;Abstract: Multimeric complexes of synaptic vesicle and terminal membrane proteins are important components of the neurotransmitter release mechanism. The csp gene of Drosophila encodes proteins homologous to synaptic vesicle proteins in Torpedo. Monoclonal antibodies demonstrate different distributions of isoforms at distinct subsets of terminals. Deletion of the csp gene in Drosophila causes a temperature-sensitive block of synaptic transmission, followed by paralysis and premature death.
- Reifegerste, R., Grimm, S., Albert, S., Lipski, N., Heimbeck, G., Hofbauer, A., Pflugfelder, G. O., Quack, D., Reichmuth, C., Schug, B., Zinsmaier, K. E., Buchner, S., & Buchner, E. (1993). An invertebrate calcium-binding protein of the calbindin subfamily: Protein structure, genomic organization, and expression pattern of the calbindin-32 gene of Drosophila. Journal of Neuroscience, 13(5), 2186-2198.More infoPMID: 8478695;Abstract: Antisera against vertebrate calcium-binding proteins cross-react with Drosophila nervous and muscle tissue. We have used an antiserum against carp parvalbumin to isolate from a Drosophila head cDNA library immunopositive expression clones. Tissue in situ hybridization identified a clone that labeled specific neurons and muscles similar to the parvalbumin-like immunohistochemical staining pattern. Five independent cDNAs derive from an mRNA whose open reading frame codes for a 310 amino acid polypeptide. Sequence analysis identifies six EF-hand calcium-binding domains and reveals 42% and 37% homology to chicken calretinin and calbindin D-28k, respectively. Since the positions of 9 out of 10 introns within the ORF are conserved from the Drosophila gene to both vertebrate genes, we conclude that we have identified the first invertebrate member of the calbindin subfamily of calcium-binding protein genes of the EF-hand homolog family. The calbindin- 32 gene (cbn) maps to 53E on the second chromosome. It is expressed through most of ontogenesis with a selective distribution in the nervous system and in a few small adult thoracic muscles. The cloning of a Drosophila homolog to vertebrate neuronal Ca2+-binding proteins opens new routes to study the so far largely elusive function of these brain molecules.
- Zinsmaier, K. E., Buchner, E., Buchner, S., Pflugfelder, G. O., Heimbeck, G., & Hofbauer, A. (1990). A Cysteine-String Protein is Expressed in Retina and Brain of Drosophila. Journal of Neurogenetics. doi:10.3109/01677069009084150More infoAntibodies can be used to identify tissue- and stage-specifically expressed genes. A monoclonal antibody MAB ab49 from a hybridoma library screened for immunohistochemical staining in the adult nervous system of Drosophila melanogaster was found to selectively bind to all neuropil regions and to synaptic boutons of motor neurons. In Western blots of homogenized brains the antibody recognizes two proteins of 32 and 34 kD. Using this antibody we have isolated seven cDNA clones that derive from two polyadenylated mRNA splice variants of a gene located at 79E1-2 on polytene chromosomes. The two mRNAs code for two inferred proteins of 249 and 223 amino acids, respectively, which are identical except for their C-terminals and a central deletion of 21 amino acids in the second protein. Both contain a contiguous string of 11 cysteine residues. In situ hybridization to frozen head sections detects expression of this gene in retina and neuronal perikarya. The 32 and 34 kD brain proteins that presumably are localized predominantly in synaptic terminals of photoreceptors and most if not all neurons may correspond to two variant cysteine-string proteins as they are of similar molecular weight and share an antigenic binding site for MAB ab49.
Presentations
- Imler, E., Pyon, J., Zhang, Y., Chandra, S., & Zinsmaier, K. E. (2015, October). A Drosophila model of CLN4B, a neurodegenerative autosomal dominant adult-onset Neuronal Ceroid Lipofuscinosis, links CSPα’s chaperone activity to toxicity.. . “Neurobiology of Drosophila” Research Conference. Cold Spring Harbor Laboratories, Sep. 29 - Oct. 3, Cold Spring Harbor, NY.
- Imler, E., Pyon, J., Zhang, Y., Chandra, S., & Zinsmaier, K. E. (2015, October). A Drosophila model of CLN4B, a neurodegenerative autosomal dominant adult-onset Neuronal Ceroid Lipofuscinosis, links CSPα’s chaperone activity to toxicity.. Annual Meeting of the “Society for Neuroscience”. Oct. 17-21, Chicago, Il.
- Zinsmaier, K. E. (2013, April). Evoked neurotransmitter release requires high levels of the anionic lipid phosphatidylserine in the cytoplasmic leaflet of the presynaptic membrane. Neurobiology Symposium “Neural Development, Function and Demise: A Genetic Perspective“. Baylor College of Medicine, Houston, TX.
- Zinsmaier, K. E. (2012, December). A presynaptic signaling role for the lipid phosphatidylserine (PS): Implications for SV exo- and endocytosis. Program in Neuroscience Seminar Series. University of Massachusetts Medical School, Worcester, MA..
- Zinsmaier, K. E. (2012, February). Mechanisms controlling the direction of mitochondrial transport and mitochondrial logistics in axons. Seminar Series Department of Biological Sciences. Florida Atlantic University, Boca Raton, FL.
- Zinsmaier, K. E., Russo, G. J., Wellington, A. J., & Babic, M. (2012, April). Miro controls mitochondrial transport, structure, and health in axons through its GTPase or EF-hand domains.. Keystone Symposia: Mitochondrial Dynamics and Function. Banff, Alberta, Canada.
Poster Presentations
- Patron, L. A., Imad, M., Nagatomo, K., & Zinsmaier, K. E. (2015, October). Drosophila WDR40A is required for evoked release and synaptic homeostasis at the neuromuscular junction.. “Neurobiology of Drosophila” Research Conference. Cold Spring Harbor Laboratories, Sep. 29 - Oct. 3, Cold Spring Harbor, NY.
- Babic, M., Hunter, B. W., Russo, G. J., Wellington, A. J., & Zinsmaier, K. E. (2014, November). Control of antero- and retrograde mitochondrial transport in Drosophila motor axons.. Annual Meeting of the Society for Neuroscience. Washington, DC.
- Patrón, L., Imad, M., Nagatomo, K., & Zinsmaier, K. E. (2014, November). Drosophila WDR40A is critical for synaptic growth and neurotransmitter release at the neuromuscular junction. Annual Meeting of the “Society for Neuroscience”. Washington, DC: Society for Neuroscience.
- Babic, M., Kuhn, T. M., & Zinsmaier, K. E. (2013, November). The conserved isoforms of Miro may have different roles for mitochondrial biology in axons of Drosophila. Annual Meeting of the Society for Neuroscience. San Diego, CA.
- Imad, M., Gay, C., Imler, E. E., Torvund, M., & Zinsmaier, K. E. (2013, November). The anionic phospholipid phosphatidylserine is involved in synaptic vesicle exo- and endocytosis at larval neuromuscular junctions. Annual Meeting of the Society for Neuroscience. San Diego, CA.
- Imler, E. E., Imad, M., Babic, M., & Zinsmaier, K. E. (2013, October). The dynamic role of the anionic lipid phosphatidylserine during the endocytosis and trafficking of synaptic vesicles. Neurobiology of Drosophila Research Conference. Cold Spring Harbor, New York.
- Patron, L. A., Imad, M., Nagatomo, K., & Zinsmaier, K. E. (2013, April). Drosophila WDR40A facilitates evoked neurotransmitter release at presynaptic terminals via a novel lipid based mechanism. 54th Annual Drosophila Research Conference. Washington, DC.
- Imad, M., Gay, C., Imler, E. E., Torvund, M., & Zinsmaier, K. E. (2012, October). dATP8B-mediated inward translocation of phosphatidylserine is required for evoked neurotransmitter release at larval neuromuscular junctions of Drosophila. 42nd Annual Meeting of the “Society for Neuroscience”. New Orleans, LA.
- Imler, E. E., Imad, M., Gay, C., & Zinsmaier, K. E. (2012, October). Does the anionic lipid phosphatidylserine mark the endo- and exocytotic route of the synaptic vesicle cycle?. 42nd Annual Meeting of the “Society for Neuroscience”. New Orleans, LA.
- Patron, L. A., Imad, M., Nagatomo, K., Babic, M., & Zinsmaier, K. E. (2012, October). Drosophila dWD40A controls synaptic growth and function in a neuron-specific manner. 42nd Annual Meeting of the “Society for Neuroscience”,. New Orleans, LA.
- Russo, G. J., Wellington, A. J., Babic, M., & Zinsmaier, K. E. (2012, April). Miro controls mitochondrial transport, structure, and health in axons through its GTPase or EF-hand domains.. Keystone Symposia: Mitochondrial Dynamics and Function. Banff, Alberta, Canada.
- Russo, G. J., Wellington, A. J., Babic, M., & Zinsmaier, K. E. (2012, June). Miro’s GTPase domains control mitochondrial transport and structure in axons of Drosophila. “Mitochondrial Trafficking and Function in Neuronal Health and Disease" Abcam Meeting. Harvard Medical School, Boston, MS.