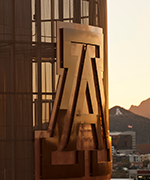
William R Montfort
- Professor, Chemistry and Biochemistry-Sci
- Professor, BIO5 Institute
- Professor, Molecular and Cellular Biology
- Professor, Applied Mathematics - GIDP
- Professor, Cancer Biology - GIDP
- Professor, Genetics - GIDP
- Member of the Graduate Faculty
- Professor, Chemistry and Biochemistry - Med
Contact
- (520) 621-1884
- Biological Sciences West, Rm. 524
- Tucson, AZ 85721
- montfort@arizona.edu
Bio
No activities entered.
Interests
No activities entered.
Courses
2024-25 Courses
-
Nucleic Acids, Metab & Signal
BIOC 568 (Spring 2025) -
Research
BIOC 900 (Spring 2025) -
Biological Structure 1
BIOC 585A (Fall 2024) -
Dissertation
BIOC 920 (Fall 2024) -
Dissertation
CBIO 920 (Fall 2024)
2023-24 Courses
-
Honors Directed Research
BIOC 492H (Summer I 2024) -
Dissertation
CBIO 920 (Spring 2024) -
Nucleic Acids, Metab & Signal
BIOC 568 (Spring 2024) -
Research
BIOC 900 (Spring 2024) -
Senior Capstone
BIOC 498 (Spring 2024) -
Biological Structure 1
BIOC 585A (Fall 2023) -
Dissertation
CBIO 920 (Fall 2023) -
Research Conference
CBIO 695A (Fall 2023) -
Seminar in Biochemistry
BIOC 696D (Fall 2023) -
Senior Capstone
BIOC 498 (Fall 2023)
2022-23 Courses
-
Directed Research
BIOC 392 (Spring 2023) -
Directed Research
MCB 792 (Spring 2023) -
Dissertation
CBIO 920 (Spring 2023) -
Nucleic Acids, Metab & Signal
BIOC 568 (Spring 2023) -
Research Conference
CBIO 695A (Spring 2023) -
Directed Research
BIOC 392 (Fall 2022) -
Dissertation
BIOC 920 (Fall 2022) -
Dissertation
CBIO 920 (Fall 2022) -
Metabolic Biochemistry
BIOC 385 (Fall 2022) -
Seminar in Biochemistry
BIOC 696D (Fall 2022)
2021-22 Courses
-
Biochemistry
BIOC 462B (Spring 2022) -
Dissertation
BIOC 920 (Spring 2022) -
Dissertation
CBIO 920 (Spring 2022) -
Introduction to Research
BIOC 792 (Spring 2022) -
Research
BIOC 900 (Spring 2022) -
Research Conference
CBIO 695A (Spring 2022) -
Dissertation
BIOC 920 (Fall 2021) -
Dissertation
CBIO 920 (Fall 2021) -
Master's Report
ABS 909 (Fall 2021) -
Research
BIOC 900 (Fall 2021) -
Research Conference
CBIO 695A (Fall 2021)
2020-21 Courses
-
Biochemistry
BIOC 462B (Spring 2021) -
Dissertation
BIOC 920 (Spring 2021) -
Dissertation
CBIO 920 (Spring 2021) -
Dissertation
CHEM 920 (Spring 2021) -
Exchange Chemical Info
CHEM 695B (Spring 2021) -
Internship in Applied Biosci
ABS 593A (Spring 2021) -
Master's Report
ABS 909 (Spring 2021) -
Research
BIOC 900 (Spring 2021) -
Research
CHEM 900 (Spring 2021) -
Research Conference
CBIO 695A (Spring 2021) -
Dissertation
BIOC 920 (Fall 2020) -
Exchange Chemical Info
CHEM 695B (Fall 2020) -
Research
CBIO 900 (Fall 2020) -
Research
CHEM 900 (Fall 2020) -
Research Conference
CBIO 695A (Fall 2020)
2019-20 Courses
-
Dissertation
BIOC 920 (Spring 2020) -
Exchange Chemical Info
CHEM 695B (Spring 2020) -
Internship in Applied Biosci
ABS 593A (Spring 2020) -
Lab Presentations+Discs
BIOC 696A (Spring 2020) -
Research
CHEM 900 (Spring 2020) -
Dissertation
BIOC 920 (Fall 2019) -
Dissertation
CHEM 920 (Fall 2019) -
Exchange Chemical Info
CHEM 695B (Fall 2019) -
Research
BIOC 900 (Fall 2019) -
Research
CHEM 900 (Fall 2019) -
Senior Capstone
BIOC 498 (Fall 2019)
2018-19 Courses
-
Independent Study
MCB 599 (Summer I 2019) -
Independent Study
MCB 699 (Summer I 2019) -
Biochemistry
BIOC 462B (Spring 2019) -
Dissertation
BIOC 920 (Spring 2019) -
Exchange Chemical Info
CHEM 695B (Spring 2019) -
Lab Presentations+Discs
BIOC 696A (Spring 2019) -
Research
BIOC 900 (Spring 2019) -
Research
CHEM 900 (Spring 2019) -
Senior Capstone
BIOC 498 (Spring 2019) -
Biological Structure 1
BIOC 585A (Fall 2018) -
Dissertation
BIOC 920 (Fall 2018) -
Exchange Chemical Info
CHEM 695B (Fall 2018) -
Introduction to Research
BIOC 795A (Fall 2018) -
Research
BIOC 900 (Fall 2018) -
Senior Capstone
BIOC 498 (Fall 2018)
2017-18 Courses
-
Biochemistry
BIOC 462B (Spring 2018) -
Directed Research
BIOC 492 (Spring 2018) -
Dissertation
BIOC 920 (Spring 2018) -
Dissertation
CHEM 920 (Spring 2018) -
Exchange Chemical Info
CHEM 695B (Spring 2018) -
Research
BIOC 900 (Spring 2018) -
Research
CHEM 900 (Spring 2018) -
Senior Capstone
BIOC 498 (Spring 2018) -
Directed Research
BIOC 492 (Fall 2017) -
Dissertation
BIOC 920 (Fall 2017) -
Exchange Chemical Info
CHEM 695B (Fall 2017) -
Introduction to Research
BIOC 795A (Fall 2017) -
Introduction to Research
MCB 795A (Fall 2017) -
Research
BIOC 900 (Fall 2017) -
Research
CHEM 900 (Fall 2017) -
Senior Capstone
BIOC 498 (Fall 2017)
2016-17 Courses
-
Biochemistry
BIOC 462B (Spring 2017) -
Directed Research
BIOC 492 (Spring 2017) -
Dissertation
BIOC 920 (Spring 2017) -
Exchange Chemical Info
CHEM 695B (Spring 2017) -
Honors Thesis
BIOC 498H (Spring 2017) -
Introduction to Research
MCB 795A (Spring 2017) -
Research
BIOC 900 (Spring 2017) -
Research
CHEM 900 (Spring 2017) -
Biochemistry
BIOC 462A (Fall 2016) -
Biological Structure 1
BIOC 585A (Fall 2016) -
Dissertation
BIOC 920 (Fall 2016) -
Exchange Chemical Info
CHEM 695B (Fall 2016) -
Honors Thesis
BIOC 498H (Fall 2016) -
Research
BIOC 900 (Fall 2016) -
Research
CHEM 900 (Fall 2016)
2015-16 Courses
-
Biochemistry
BIOC 462B (Spring 2016) -
Directed Research
BIOC 492 (Spring 2016) -
Dissertation
BIOC 920 (Spring 2016) -
Exchange Chemical Info
CHEM 695B (Spring 2016) -
Honors Preceptorship
BIOC 491H (Spring 2016) -
Lab Presentations+Discs
BIOC 696A (Spring 2016) -
Research
BIOC 900 (Spring 2016)
Scholarly Contributions
Journals/Publications
- Wales, J. A., Chen, C. Y., Breci, L., Weichsel, A., Bernier, S. G., Sheppeck, J. E., Solinga, R., Nakai, T., Renhowe, P. A., Jung, J., & Montfort, W. R. (2018). Discovery of stimulator binding to a conserved pocket in the heme domain of soluble guanylyl cyclase. The Journal of biological chemistry, 293(5), 1850-1864.More infoSoluble guanylyl cyclase (sGC) is the receptor for nitric oxide and a highly sought-after therapeutic target for the management of cardiovascular diseases. New compounds that stimulate sGC show clinical promise, but where these stimulator compounds bind and how they function remains unknown. Here, using a photolyzable diazirine derivative of a novel stimulator compound, IWP-051, and MS analysis, we localized drug binding to the β1 heme domain of sGC proteins from the hawkmothand from human. Covalent attachments to the stimulator were also identified in bacterial homologs of the sGC heme domain, referred to as H-NOX domains, including those fromsp. PCC 7120,,, and, indicating that the binding site is highly conserved. The identification of photoaffinity-labeled peptides was aided by a signature MS fragmentation pattern of general applicability for unequivocal identification of covalently attached compounds. Using NMR, we also examined stimulator binding to sGC fromand bacterial H-NOX homologs. These data indicated that stimulators bind to a conserved cleft between two subdomains in the sGC heme domain. L12W/T48W substitutions within the binding pocket resulted in a 9-fold decrease in drug response, suggesting that the bulkier tryptophan residues directly block stimulator binding. The localization of stimulator binding to the sGC heme domain reported here resolves the longstanding question of where stimulators bind and provides a path forward for drug discovery.
- Montfort, W. R., Wales, J. A., & Weichsel, A. (2017). Structure and Activation of Soluble Guanylyl Cyclase, the Nitric Oxide Sensor. Antioxidants & redox signaling, 26(3), 107-121.More infoSoluble guanylyl/guanylate cyclase (sGC) is the primary receptor for nitric oxide (NO) and is central to the physiology of blood pressure regulation, wound healing, memory formation, and other key physiological activities. sGC is increasingly implicated in disease and is targeted by novel therapeutic compounds. The protein displays a rich evolutionary history and a fascinating signal transduction mechanism, with NO binding to an N-terminal heme-containing domain, which activates the C-terminal cyclase domains. Recent Advances: Crystal structures of individual sGC domains or their bacterial homologues coupled with small-angle x-ray scattering, electron microscopy, chemical cross-linking, and Förster resonance energy transfer measurements are yielding insight into the overall structure for sGC, which is elongated and likely quite dynamic. Transient kinetic measurements reveal a role for individual domains in lowering NO affinity for heme. New sGC stimulatory drugs are now in the clinic and appear to function through binding near or directly to the sGC heme domain, relieving inhibitory contacts with other domains. New sGC-activating drugs show promise for recovering oxidized sGC in diseases with high inflammation by replacing lost heme.
- Berry, R. E., Yang, F., Shokhireva, T. K., Amoia, A. M., Garrett, S. A., Goren, A. M., Korte, S. R., Zhang, H., Weichsel, A., Montfort, W. R., & Walker, F. A. (2015). Dimerization of nitrophorin 4 at low pH and comparison to the K1A mutant of nitrophorin 1. Biochemistry, 54(2), 208-20.More infoNitrophorin 4, one of the four NO-carrying heme proteins from the salivary glands of Rhodnius prolixus, forms a homodimer at pH 5.0 with a Kd of ∼8 μM. This dimer begins to dissociate at pH 5.5 and is completely dissociated to monomer at pH 7.3, even at 3.7 mM. The dimer is significantly stabilized by binding NO to the heme and at pH 7.3 would require dilution to well below 0.2 mM to completely dissociate the NP4-NO homodimer. The primary techniques used for investigating the homodimer and the monomer-dimer equilibrium were size-exclusion fast protein liquid chromatography at pH 5.0 and (1)H{(15)N} heteronuclear single-quantum coherence spectroscopy as a function of pH and concentration. Preparation of site-directed mutants of NP4 (A1K, D30A, D30N, V36A/D129A/L130A, K38A, R39A, K125A, K125E, D132A, L133V, and K38Q/R39Q/K125Q) showed that the N-terminus, D30, D129, D132, at least one heme propionate, and, by association, likely also E32 and D35 are involved in the dimerization. The "closed loop" form of the A-B and G-H flexible loops of monomeric NP4, which predominates in crystal structures of the monomeric protein reported at pH 5.6 but not at pH 7.5 and which involves all of the residues listed above except D132, is required for dimer formation. Wild-type NP1 does not form a homodimer, but NP1(K1A) and native N-terminal NP1 form dimers in the presence of NO. The homodimer of NP1, however, is considerably less stable than that of NP4 in the absence of NO. This suggests that additional aspartate or glutamate residues present in the C-terminal region of NP4, but not NP1, are also involved in stabilizing the dimer.
- Sarkar, A., Dai, Y., Haque, M. M., Seeger, F., Ghosh, A., Garcin, E. D., Montfort, W. R., Hazen, S. L., Misra, S., & Stuehr, D. J. (2015). Heat Shock Protein 90 Associates with the Per-Arnt-Sim Domain of Heme-free Soluble Guanylate Cyclase: IMplications for Enzyme Maturation. The Journal of biological chemistry, 290(35), 21615-28.More infoHeat shock protein 90 (hsp90) drives heme insertion into the β1 subunit of soluble guanylate cyclase (sGC) β1, which enables it to associate with a partner sGCα1 subunit and mature into a nitric oxide (NO)-responsive active form. We utilized fluorescence polarization measurements and hydrogen-deuterium exchange mass spectrometry to define molecular interactions between the specific human isoforms hsp90β and apo-sGCβ1. hsp90β and its isolated M domain, but not its isolated N and C domains, bind with low micromolar affinity to a heme-free, truncated version of sGCβ1 (sGCβ1(1-359)-H105F). Surprisingly, hsp90β and its M domain bound to the Per-Arnt-Sim (PAS) domain of apo-sGC-β1(1-359), which lies adjacent to its heme-binding (H-NOX) domain. The interaction specifically involved solvent-exposed regions in the hsp90β M domain that are largely distinct from sites utilized by other hsp90 clients. The interaction strongly protected two regions of the sGCβ1 PAS domain and caused local structural relaxation in other regions, including a PAS dimerization interface and a segment in the H-NOX domain. Our results suggest a means by which the hsp90β interaction could prevent apo-sGCβ1 from associating with its partner sGCα1 subunit while enabling structural changes to assist heme insertion into the H-NOX domain. This mechanism would parallel that in other clients like the aryl hydrocarbon receptor and HIF1α, which also interact with hsp90 through their PAS domains to control protein partner and small ligand binding interactions.
- Gloss, A. D., Vassão, D. G., Hailey, A. L., Nelson Dittrich, A. C., Schramm, K., Reichelt, M., Rast, T. J., Weichsel, A., Cravens, M. G., Gershenzon, J., Montfort, W. R., & Whiteman, N. K. (2014). Evolution in an ancient detoxification pathway is coupled with a transition to herbivory in the drosophilidae. Molecular biology and evolution, 31(9), 2441-56.More infoChemically defended plant tissues present formidable barriers to herbivores. Although mechanisms to resist plant defenses have been identified in ancient herbivorous lineages, adaptations to overcome plant defenses during transitions to herbivory remain relatively unexplored. The fly genus Scaptomyza is nested within the genus Drosophila and includes species that feed on the living tissue of mustard plants (Brassicaceae), yet this lineage is derived from microbe-feeding ancestors. We found that mustard-feeding Scaptomyza species and microbe-feeding Drosophila melanogaster detoxify mustard oils, the primary chemical defenses in the Brassicaceae, using the widely conserved mercapturic acid pathway. This detoxification strategy differs from other specialist herbivores of mustard plants, which possess derived mechanisms to obviate mustard oil formation. To investigate whether mustard feeding is coupled with evolution in the mercapturic acid pathway, we profiled functional and molecular evolutionary changes in the enzyme glutathione S-transferase D1 (GSTD1), which catalyzes the first step of the mercapturic acid pathway and is induced by mustard defense products in Scaptomyza. GSTD1 acquired elevated activity against mustard oils in one mustard-feeding Scaptomyza species in which GstD1 was duplicated. Structural analysis and mutagenesis revealed that substitutions at conserved residues within and near the substrate-binding cleft account for most of this increase in activity against mustard oils. Functional evolution of GSTD1 was coupled with signatures of episodic positive selection in GstD1 after the evolution of herbivory. Overall, we found that preexisting functions of generalized detoxification systems, and their refinement by natural selection, could play a central role in the evolution of herbivory.
- Montfort, W. R. (2016). Structure and Activation of Soluble Guanylyl Cyclase, the Nitric Oxide Sensor. Antioxid. Redox. Signal..
- Purohit, R., Fritz, B. G., The, J., Issaian, A., Weichsel, A., David, C. L., Campbell, E., Hausrath, A. C., Rassouli-Taylor, L., Garcin, E. D., Gage, M. J., & Montfort, W. R. (2014). YC-1 binding to the β subunit of soluble guanylyl cyclase overcomes allosteric inhibition by the α subunit. Biochemistry, 53(1), 101-114.More infoPMID: 24328155;Abstract: Soluble guanylate cyclase (sGC) is a heterodimeric heme protein and the primary nitric oxide receptor. NO binding stimulates cyclase activity, leading to regulation of cardiovascular physiology and making sGC an attractive target for drug discovery. YC-1 and related compounds stimulate sGC both independently and synergistically with NO and CO binding; however, where the compounds bind and how they work remain unknown. Using linked equilibrium binding measurements, surface plasmon resonance, and domain truncations in Manduca sexta and bovine sGC, we demonstrate that YC-1 binds near or directly to the heme-containing domain of the β subunit. In the absence of CO, YC-1 binds with a K d of 9-21 μM, depending on the construct. In the presence of CO, these values decrease to 0.6-1.1 μM. Pfizer compound 25 bound ∼10-fold weaker than YC-1 in the absence of CO, whereas compound BAY 41-2272 bound particularly tightly in the presence of CO (Kd = 30-90 nM). Additionally, we found that CO binds much more weakly to heterodimeric sGC proteins (Kd = 50-100 μM) than to the isolated heme domain (K d = 0.2 μM for Manduca β H-NOX/PAS). YC-1 greatly enhanced binding of CO to heterodimeric sGC, as expected (Kd ∼ 1 μM). These data indicate the α subunit induces a heme pocket conformation with a lower affinity for CO and NO. YC-1 family compounds bind near the heme domain, overcoming the α subunit effect and inducing a heme pocket conformation with high affinity. We propose this high-affinity conformation is required for the full-length protein to achieve high catalytic activity. © 2013 American Chemical Society.
- Cheng, M., Brookes, J. F., Montfort, W. R., & Khalil, M. (2013). pH-dependent picosecond structural dynamics in the distal pocket of nitrophorin 4 investigated by 2D IR spectroscopy. The journal of physical chemistry. B, 117(49), 15804-11.More infoNitrophorin 4 (NP4) belongs to a family of pH-sensitive, nitric oxide (NO) transporter proteins that undergo a large structural change from a closed to an open conformation at high pH to allow for NO delivery. Measuring the pH-dependent structural dynamics in NP4-NO around the ligand binding site is crucial for developing a mechanistic understanding of NO binding and release. In this study, we use coherent two-dimensional infrared (2D IR) spectroscopy to measure picosecond structural dynamics sampled by the nitrosyl stretch in NP4-NO as a function of pH at room temperature. Our results show that both the closed and open conformers of the protein are present at low (pD 5.1) and high (pD 7.9) pH conditions. The closed and open conformers are characterized by two frequencies of the nitrosyl stretching vibration labeled A0 and A1, respectively. Analysis of the 2D IR line shapes reveals that at pD 5.1, the closed conformer experiences structural fluctuations arising from solvation dynamics on a ∼3 ps time scale. At pD 7.9, both the open and closed conformers exhibit fluctuations on a ∼1 ps time scale. At both pD conditions, the closed conformers maintain a static distribution of structures within the experimental time window of 100 ps. This is in contrast to the open conformer, which is able to interconvert among its substates on a ∼100 ps time scale. Our results directly measure the time scales of solvation dynamics in the distal pocket, the flexibility of the open conformation at high pH, and the rigidity of the closed conformers at both pH conditions. We discuss how the pH-dependent equilibrium structural fluctuations of the nitrosyl ligand measured in this study are related to the uptake and delivery of nitric oxide in NP4.
- Fritz, B. G., Roberts, S. A., Ahmed, A., Breci, L., Li, W., Weichsel, A., Brailey, J. L., Wysocki, V. H., Tama, F., & Montfort, W. R. (2013). Molecular model of a soluble guanylyl cyclase fragment determined by small-angle X-ray scattering and chemical cross-linking. Biochemistry, 52(9), 1568-82.More infoSoluble guanylyl/guanylate cyclase (sGC) converts GTP to cGMP after binding nitric oxide, leading to smooth muscle relaxation and vasodilation. Impaired sGC activity is common in cardiovascular disease, and sGC stimulatory compounds are vigorously sought. sGC is a 150 kDa heterodimeric protein with two H-NOX domains (one with heme, one without), two PAS domains, a coiled-coil domain, and two cyclase domains. Binding of NO to the sGC heme leads to proximal histidine release and stimulation of catalytic activity. To begin to understand how binding leads to activation, we examined truncated sGC proteins from Manduca sexta (tobacco hornworm) that bind NO, CO, and stimulatory compound YC-1 but lack the cyclase domains. We determined the overall shape of truncated M. sexta sGC using analytical ultracentrifugation and small-angle X-ray scattering (SAXS), revealing an elongated molecule with dimensions of 115 Å × 90 Å × 75 Å. Binding of NO, CO, or YC-1 had little effect on shape. Using chemical cross-linking and tandem mass spectrometry, we identified 20 intermolecular contacts, allowing us to fit homology models of the individual domains into the SAXS-derived molecular envelope. The resulting model displays a central parallel coiled-coil platform upon which the H-NOX and PAS domains are assembled. The β1 H-NOX and α1 PAS domains are in contact and form the core signaling complex, while the α1 H-NOX domain can be removed without a significant effect on ligand binding or overall shape. Removal of 21 residues from the C-terminus yields a protein with dramatically increased proximal histidine release rates upon NO binding.
- Purohit, R., Weichsel, A., & Montfort, W. R. (2013). Crystal structure of the Alpha subunit PAS domain from soluble guanylyl cyclase. Protein science : a publication of the Protein Society, 22(10), 1439-44.More infoSoluble guanylate cyclase (sGC) is a heterodimeric heme protein of ≈ 150 kDa and the primary nitric oxide receptor. Binding of NO stimulates cyclase activity, leading to regulation of cardiovascular physiology and providing attractive opportunities for drug discovery. How sGC is stimulated and where candidate drugs bind remains unknown. The α and β sGC chains are each composed of Heme-Nitric Oxide Oxygen (H-NOX), Per-ARNT-Sim (PAS), coiled-coil and cyclase domains. Here, we present the crystal structure of the α1 PAS domain to 1.8 Å resolution. The structure reveals the binding surfaces of importance to heterodimer function, particularly with respect to regulating NO binding to heme in the β1 H-NOX domain. It also reveals a small internal cavity that may serve to bind ligands or participate in signal transduction.
- Xu, Y., Zhou, T., Zhou, Z., Su, S., Roberts, S. A., Montfort, W. R., Zeng, J., Chen, M., Zhang, W., Lin, M., Zhan, J., & Molnár, I. (2013). Rational reprogramming of fungal polyketide first-ring cyclization. Proceedings of the National Academy of Sciences of the United States of America, 110(14), 5398-403.More infoResorcylic acid lactones and dihydroxyphenylacetic acid lactones represent important pharmacophores with heat shock response and immune system modulatory activities. The biosynthesis of these fungal polyketides involves a pair of collaborating iterative polyketide synthases (iPKSs): a highly reducing iPKS with product that is further elaborated by a nonreducing iPKS (nrPKS) to yield a 1,3-benzenediol moiety bridged by a macrolactone. Biosynthesis of unreduced polyketides requires the sequestration and programmed cyclization of highly reactive poly-β-ketoacyl intermediates to channel these uncommitted, pluripotent substrates to defined subsets of the polyketide structural space. Catalyzed by product template (PT) domains of the fungal nrPKSs and discrete aromatase/cyclase enzymes in bacteria, regiospecific first-ring aldol cyclizations result in characteristically different polyketide folding modes. However, a few fungal polyketides, including the dihydroxyphenylacetic acid lactone dehydrocurvularin, derive from a folding event that is analogous to the bacterial folding mode. The structural basis of such a drastic difference in the way a PT domain acts has not been investigated until now. We report here that the fungal vs. bacterial folding mode difference is portable on creating hybrid enzymes, and we structurally characterize the resulting unnatural products. Using structure-guided active site engineering, we unravel structural contributions to regiospecific aldol condensations and show that reshaping the cyclization chamber of a PT domain by only three selected point mutations is sufficient to reprogram the dehydrocurvularin nrPKS to produce polyketides with a fungal fold. Such rational control of first-ring cyclizations will facilitate efforts to the engineered biosynthesis of novel chemical diversity from natural unreduced polyketides.
Poster Presentations
- Restifo, L. L., Andrew, D. R., Johnstone, L. M., Miller, J. H., Montfort, W. R., Day, W. A., Hammer, M. F., & Scherer, K. (2021, November). A sporadic case of severe peripheral neuropathy expands the phenotypic range of TUBB2A mutations.. Society for Neuroscience 2021.More infoWhole-exome sequencing study of patient and parents revealed a de novo mutation in TUBB2A which is predicted, based on 3D structure modeling, to disrupt the contact site between beta-tubulin and kinesin. This would explain the proband's childhood-onset severe sensorimotor peripheral neuropathy.The figures were finalized for poster presentation at the (virtual) Society Neuroscience conference in Nov. 2021. We're preparing the manuscript for Frontiers in Neurology and hope to submit in Spring 2022.