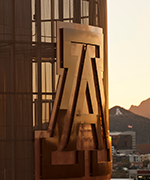
Jon T Njardarson
- Professor, Chemistry and Biochemistry-Sci
- Professor, BIO5 Institute
- Member of the Graduate Faculty
Contact
- (520) 626-0754
- Carl S. Marvel Labs of Chem., Rm. 442
- Tucson, AZ 85721
- njardars@arizona.edu
Degrees
- Ph.D. Chemistry
- Yale Chemistry, New Haven, Connecticut, United States
- B.S. Chemistry
- University of Iceland, Reykjavik, Iceland
Work Experience
- University of Arizona, Tucson, Arizona (2010 - Ongoing)
- MSKCC, Weill-Cornell and Rockefeller University (2005 - 2010)
- Cornell University, Ithaca, New York (2004 - 2010)
- Memorial Sloan-Kettering Cancer Center (MSKCC) (2001 - 2004)
Awards
- Helgu Jonsdottir and Sigurlida Kristjansson Memorial Foundation Award
- Helgu Jonsdottir and Sigurlida Kristjansson Memorial Foundation, Spring 1995 (Award Finalist)
- Distinguished Scholar Award
- University of Arizona, Spring 2016 (Award Finalist)
- Top Reviewer
- Wiley-VCH, Spring 2016
- Wiley-VCH, Spring 2015
- Wiley-VCH, Spring 2014
- Top 10 Reviewer
- Tetrahedron (Elsevier), Spring 2012
- Tetrahedron Letters (Elsevier), Spring 2012
- Merrill Presidential Scholar Outstanding Educator
- Cornell University, Spring 2009 (Award Finalist)
- Thieme Chemistry Journal Award
- Thieme publishers, Fall 2008 (Award Finalist)
- Marilyn Emmons Williams Award
- Cornell University, Spring 2006 (Award Finalist)
- General Motors Cancer Research Scholar Program Award
- General Motors Cancer Research Scholar Program, Fall 2001 (Award Finalist)
Interests
No activities entered.
Courses
2024-25 Courses
-
Dissertation
CHEM 920 (Fall 2024) -
Exchange Chemical Info
CHEM 695B (Fall 2024) -
Lecture Organic Chem
CHEM 241A (Fall 2024) -
Research
CHEM 900 (Fall 2024)
2023-24 Courses
-
Directed Research
CHEM 392 (Spring 2024) -
Directed Research
CHEM 492 (Spring 2024) -
Directed Research
ECOL 492 (Spring 2024) -
Dissertation
CHEM 920 (Spring 2024) -
Exchange Chemical Info
CHEM 695B (Spring 2024) -
Honors Directed Research
CHEM 392H (Spring 2024) -
Honors Independent Study
PSIO 499H (Spring 2024) -
Honors Thesis
BIOC 498H (Spring 2024) -
Research
CHEM 900 (Spring 2024) -
Senior Capstone
BIOC 498 (Spring 2024) -
Directed Research
CHEM 392 (Fall 2023) -
Directed Research
CHEM 492 (Fall 2023) -
Directed Research
ECOL 492 (Fall 2023) -
Dissertation
CHEM 920 (Fall 2023) -
Exchange Chemical Info
CHEM 695B (Fall 2023) -
Honors Directed Research
BIOC 392H (Fall 2023) -
Honors Directed Research
CHEM 392H (Fall 2023) -
Honors Independent Study
PSIO 399H (Fall 2023) -
Honors Preceptorship
CHEM 491H (Fall 2023) -
Honors Thesis
BIOC 498H (Fall 2023) -
Lecture Organic Chem
CHEM 241A (Fall 2023) -
Preceptorship
CHEM 491 (Fall 2023) -
Research
CHEM 900 (Fall 2023) -
Senior Capstone
BIOC 498 (Fall 2023)
2022-23 Courses
-
Directed Research
BIOC 392 (Spring 2023) -
Directed Research
BIOC 492 (Spring 2023) -
Directed Research
CHEM 392 (Spring 2023) -
Directed Research
CHEM 492 (Spring 2023) -
Dissertation
CHEM 920 (Spring 2023) -
Exchange Chemical Info
CHEM 695B (Spring 2023) -
Honors Directed Research
BIOC 392H (Spring 2023) -
Honors Independent Study
MCB 399H (Spring 2023) -
Honors Thesis
BIOC 498H (Spring 2023) -
Research
CHEM 900 (Spring 2023) -
Directed Research
BIOC 392 (Fall 2022) -
Directed Research
BIOC 492 (Fall 2022) -
Directed Research
CHEM 392 (Fall 2022) -
Directed Research
CHEM 492 (Fall 2022) -
Dissertation
CHEM 920 (Fall 2022) -
Exchange Chemical Info
CHEM 695B (Fall 2022) -
Honors Preceptorship
CHEM 491H (Fall 2022) -
Honors Thesis
BIOC 498H (Fall 2022) -
Introduction to Research
BIOC 792 (Fall 2022) -
Lecture Organic Chem
CHEM 241A (Fall 2022) -
Preceptorship
CHEM 491 (Fall 2022) -
Research
CHEM 900 (Fall 2022)
2021-22 Courses
-
Dissertation
CHEM 920 (Spring 2022) -
Exchange Chemical Info
CHEM 695B (Spring 2022) -
Research
CHEM 900 (Spring 2022) -
Dissertation
CHEM 920 (Fall 2021) -
Dissertation
PHSC 920 (Fall 2021) -
Exchange Chemical Info
CHEM 695B (Fall 2021) -
Lecture Organic Chem
CHEM 241A (Fall 2021) -
Preceptorship
CHEM 291 (Fall 2021) -
Preceptorship
CHEM 491 (Fall 2021) -
Research
CHEM 900 (Fall 2021)
2020-21 Courses
-
Dissertation
CHEM 920 (Spring 2021) -
Dissertation
PHSC 920 (Spring 2021) -
Exchange Chemical Info
CHEM 695B (Spring 2021) -
Research
CHEM 900 (Spring 2021) -
Adv Organic Synthesis
CHEM 640 (Fall 2020) -
Dissertation
CHEM 920 (Fall 2020) -
Dissertation
PHSC 920 (Fall 2020) -
Exchange Chemical Info
CHEM 695B (Fall 2020) -
Lec in Organic Chemistry
CHEM 246A (Fall 2020) -
Organic Chemistry
CHEM 696C (Fall 2020) -
Research
CHEM 900 (Fall 2020)
2019-20 Courses
-
Directed Research
BIOC 392 (Spring 2020) -
Dissertation
CHEM 920 (Spring 2020) -
Dissertation
PHSC 920 (Spring 2020) -
Exchange Chemical Info
CHEM 695B (Spring 2020) -
Organic Chemistry
CHEM 696C (Spring 2020) -
Research
CHEM 900 (Spring 2020) -
Research
PHSC 900 (Spring 2020) -
Research Conference
PCOL 695A (Spring 2020) -
Dissertation
CHEM 920 (Fall 2019) -
Dissertation
PHSC 920 (Fall 2019) -
Exchange Chemical Info
CHEM 695B (Fall 2019) -
Honors Preceptorship
CHEM 491H (Fall 2019) -
Lec in Organic Chemistry
CHEM 246A (Fall 2019) -
Organic Chemistry
CHEM 696C (Fall 2019) -
Preceptorship
CHEM 291 (Fall 2019) -
Preceptorship
CHEM 491 (Fall 2019) -
Research
CHEM 900 (Fall 2019) -
Research Conference
PCOL 695A (Fall 2019)
2018-19 Courses
-
Lecture Organic Chem
CHEM 241A (Summer I 2019) -
Dissertation
CHEM 920 (Spring 2019) -
Dissertation
PHSC 920 (Spring 2019) -
Exchange Chemical Info
CHEM 695B (Spring 2019) -
Honors Independent Study
MCB 499H (Spring 2019) -
Organic Chemistry
CHEM 696C (Spring 2019) -
Research
CHEM 900 (Spring 2019) -
Research
PHSC 900 (Spring 2019) -
Senior Capstone
BIOC 498 (Spring 2019) -
Adv Organic Synthesis
CHEM 640 (Fall 2018) -
Directed Research
CHEM 392 (Fall 2018) -
Dissertation
CHEM 920 (Fall 2018) -
Exchange Chemical Info
CHEM 695B (Fall 2018) -
Honors Directed Research
CHEM 392H (Fall 2018) -
Honors Preceptorship
CHEM 491H (Fall 2018) -
Lec in Organic Chemistry
CHEM 246A (Fall 2018) -
Organic Chemistry
CHEM 696C (Fall 2018) -
Preceptorship
CHEM 291 (Fall 2018) -
Preceptorship
CHEM 491 (Fall 2018) -
Research
CHEM 900 (Fall 2018) -
Research
PHSC 900 (Fall 2018) -
Research Conference
PCOL 695A (Fall 2018) -
Senior Capstone
BIOC 498 (Fall 2018)
2017-18 Courses
-
Lecture Organic Chem
CHEM 241A (Summer I 2018) -
Directed Research
BIOC 492 (Spring 2018) -
Directed Research
CHEM 392 (Spring 2018) -
Directed Research
CHEM 492 (Spring 2018) -
Dissertation
CHEM 920 (Spring 2018) -
Exchange Chemical Info
CHEM 695B (Spring 2018) -
Organic Chemistry
CHEM 696C (Spring 2018) -
Research
CHEM 900 (Spring 2018) -
Research Conference
PCOL 695A (Spring 2018) -
Thesis
CHEM 910 (Spring 2018) -
Directed Research
BIOC 492 (Fall 2017) -
Directed Research
CHEM 492 (Fall 2017) -
Dissertation
CHEM 920 (Fall 2017) -
Exchange Chemical Info
CHEM 695B (Fall 2017) -
Lec in Organic Chemistry
CHEM 246A (Fall 2017) -
Organic Chemistry
CHEM 696C (Fall 2017) -
Preceptorship
CHEM 491 (Fall 2017) -
Research
CHEM 900 (Fall 2017) -
Research
PHSC 900 (Fall 2017) -
Research Conference
PCOL 695A (Fall 2017)
2016-17 Courses
-
Lecture Organic Chem
CHEM 241A (Summer I 2017) -
Directed Research
CHEM 492 (Spring 2017) -
Dissertation
CHEM 920 (Spring 2017) -
Exchange Chemical Info
CHEM 695B (Spring 2017) -
Organic Chemistry
CHEM 696C (Spring 2017) -
Research
CHEM 900 (Spring 2017) -
Research
PHSC 900 (Spring 2017) -
Directed Research
BIOC 392 (Fall 2016) -
Directed Research
CHEM 392 (Fall 2016) -
Directed Research
CHEM 492 (Fall 2016) -
Dissertation
CHEM 920 (Fall 2016) -
Exchange Chemical Info
CHEM 695B (Fall 2016) -
Honors Preceptorship
CHEM 491H (Fall 2016) -
Honors Thesis
BIOC 498H (Fall 2016) -
Lecture Organic Chem
CHEM 241A (Fall 2016) -
Organic Chemistry
CHEM 696C (Fall 2016) -
Preceptorship
CHEM 291 (Fall 2016) -
Preceptorship
CHEM 491 (Fall 2016) -
Research
CHEM 900 (Fall 2016) -
Research
PHSC 900 (Fall 2016)
2015-16 Courses
-
Lecture Organic Chem
CHEM 241A (Summer I 2016) -
Directed Research
BIOC 492 (Spring 2016) -
Dissertation
CHEM 920 (Spring 2016) -
Exchange Chemical Info
CHEM 695B (Spring 2016) -
Honors Thesis
BIOC 498H (Spring 2016) -
Honors Thesis
PSIO 498H (Spring 2016) -
Research
CHEM 900 (Spring 2016) -
Senior Capstone
BIOC 498 (Spring 2016)
Scholarly Contributions
Chapters
- Njardarson, J. T. (2018). Analysis of US FDA-Approved Drugs Containing Sulfur Atoms. In Topics in Current Chemistry – Sulfur Chemistry(pp 1-34). Springer.
Journals/Publications
- Njardarson, J. T. (2017). Dearomatization Approach to 2-Trifluoromethylated Benzofuran and Dihydrobenzofuran Products. Organic Letters, 19, 3508-3511.
- Njardarson, J. T. (2017). Double-Diels-Alder Approach to Maeocrystal V. Unexpected C-C Bond Forming Fragmentations of the [2.2.2]-Bicyclic Core. Organic Letters, 19, 5316-5319.
- Njardarson, J. T. (2017). New Class of Anion-Accelerated Amino-Cope Rearrangement as Gateway to Diverse Chiral Structures. Journal of the American Chemical Society, 139, 13141-13146.
- Njardarson, J. T., & Das, P. (2016). Anionic Cascade Routes to Sulfur and Nitrogen Heterocycles Originating from Thio- and Aminophosphate Precursors. European Journal of Organic Chemistry, 4249–4259. doi:10.1002/ejoc.201600312
- Njardarson, J. T., & Draghici, C. (2015). Synthetic Approaches and Total Syntheses of Vinigrol, A Unique Diterpenoid. Tetrahedron, 71, 3775-3793. doi:10.1016/j.tet.2015.04.073More infoThe aim of this review is to present a detailed account of all published total syntheses and synthetic approaches toward the unique diterpenoid natural product vinigrol. We have chosen to summarize the efforts of each paper by graphically detailing the synthesis, and associated reaction details, of only the most advanced intermediate each research team made. This review1 omits contributions only reported in graduate student theses. The remarkable structure of vinigrol, which is most simply described as a densely decorated cis-decalin core with a diaxial four carbon bridge connecting the two cyclohexyl rings, was first reported in 1987. 2 Vinigrol contains seven challenging stereocenters and is decorated with only three heteroatoms in the form of hydroxyl groups (primary, secondary and tertiary) all of which are located on one of the two cyclohexyl rings. Vinigrol has been reported to exhibit a promising biological profile, most notably in the areas of cancer and HIV.3 Shown in Scheme 1 is a compilation of all the vinigrol structural perspectives reported to date by the research teams whose efforts are summarized in the following sections. Each one of these perspectives will appear in later schemes as we utilize each authors chosen vinigrol perspective to highlight (with color) how far their synthetic efforts advanced.
- Njardarson, J. T., & Vitaku, E. (2016). A Mild meta-Selective C–H Alkylation of Catechol Mono-Ethers. European Journal of Organic Chemistry, 3679–3683. doi:10.1002/ejoc.201600760
- Njardarson, J. T., Vitaku, E., & Smith, D. T. (2016). Metal-Free Synthesis of Fluorinated Indoles Enabled by Oxidative Dearomatization. Angewandte Chemie International Edition, 55, 2243–2247. doi:10.1002/anie.201511149More infoNitrogen heterocycles are found in a majority of approved small-molecule pharmaceuticals, and the number of approved fluorinated drugs is increasing each decade. Therefore, new approaches for accessing fluorinated nitrogen heterocycles are of great significance. A novel, scalable, and metal-free method for accessing a wide range of fluorinated indoles is described. This oxidative-dearomatization-enabled approach assembles 2-trifluoromethyl NH-indole products from simple commercially available anilines with hexafluoroacetylacetone in the presence of an organic oxidant. The nature of the aniline N-capping group is critical for the success of this new reaction. Furthermore, the indole products contain a 3-trifluoroacetyl group, which can be exploited to access a plethora of useful functional groups.
- Njardarson, J. T., & Chogii, I. (2015). Asymmetric [3+2] Annulation Approach to 3-Pyrrolines. Concise Total Syntheses of (-)-Supinidine, (-)-Isoretronecanol and (+)-Elacomine. Angewandte Chemie International Edition, 54, 13706-137. doi:10.1002/anie.201506559More infoAn asymmetric [3+2] annulation reaction to form 3-pyrroline products is reported. Upon treatment with lithium diisopropylamide, readily available ethyl 4-bromocrotonate is deprotonated and trapped with Ellman imines selectively at the α-position to yield enantiopure 3-pyrroline products. This new method is compatible with aryl, alkyl, and vinyl imines. The efficacy of the method is showcased by short asymmetric total syntheses of (−)-supinidine, (−)-isoretronecanol, and (+)-elacomine. This novel annulation approach also works for an aldehyde, thus providing access to a 2,5-dihydrofuran product in a single step from simple precursors. By modifying the structure of the carbanion nucleophile, an asymmetric vinylogous aza-Darzens reaction can be realized.
- Njardarson, J. T., & Das, P. (2015). Synthesis of 1,2,3,6-Tetrahydropyridines via Aminophosphate Enabled Anionic Cascade and Acid Catalyzed Cyclization Approaches. Organic Letters, 17, 4030-4033. doi:10.1021/acs.orglett.5b01937More infoTwo new approaches for forming 1,2,3,6-tetrahydropyridines are reported. Both reactions employ a strategic phosphate substituent on the nitrogen atom. In the presence of an additional phosphate substituent (X = P = O(OEt)2) an anionic cascade can by triggered upon treatment with base. Alternatively, when X = H the same 1,2,3,6-tetrahydropyridine product can be accessed via an acid catalyzed cyclization.
- Njardarson, J. T., & Draghici, C. (2015). The Realization of an Oxidative Dearomatization-Intramolecular Diels-Alder Route to Vinigrol. Strategies and Tactics in Organic Synthesis 11, 11, 335-376.More infoDetailed in this chapter is the Njardarson group vinigrol total synthesis saga. Even though the Njardarson group synthetic strategy had undergone multiple modifications over time, it remained faithful to several key reaction design elements, namely: (a) oxidative dearomatization/Diels–Alder cascade, (b) radical or palladium cascade, (c) creation of a tetracyclic cage for substrate control purposes, and (d) a late-stage Csingle bondC bond fragmentation reaction to unravel the vinigrol core. The saga begins with the earliest oxidative dearomatization explorations followed by detailed descriptions of the modifications that were made in response to the obstacles encountered en route to the completed total synthesis of vinigrol.
- Njardarson, J. T., & Smith, D. T. (2015). Ring Expansions of Oxiranes and Oxetanes. Topics in Heterocyclic Chemistry, 41, 281-309. doi:10.1007/7081_2015_147More infoThis chapter is focused on recently published (2006–2014) oxirane and oxetane ring-expansion reactions. The majority of these advances employ oxirane substrates, the discussion of which is broken into five thematic sections representing insertions, cascades, metal-free, metal-catalyzed, and miscellaneous strategies. Oxetane ring-expansion reactions are still rare and are discussed together in one section. A concise graphical summary has been created for every new contribution to this area, highlighting reagents, reaction conditions, scope, mechanistic proposals, and asymmetry where applicable. This review chapter should enable researchers new to the field to quickly assess the current state of the art.
- Njardarson, J. T., & Vitaku, E. (2015). Formation of Fused Aromatic Architectures via an Oxidative Dearomatization - Radical Cyclization Rearomatization Approach. Tetrahedron Letters, 56, 3550-3552. doi:10.1016/j.tetlet.2015.01.110More infoA new mild C–C bond forming cyclization approach of catechol derivatives is reported. This approach relies on an initial dearomatization step using lead(IV) acetate followed by a carefully controlled radical cyclization step, which under the reaction conditions also facilitates rearomatization. Triethylborane is key to the success of this reaction as it enables the reaction to proceed at low temperatures and is also believed to aid rearomatization. The amount and ratio of triethylborane and reducing agent (tributyltinhydride) that is employed as well as the concentration the reaction is run at are all essential to the success of this new approach.
- Guo, B., Vitaku, E., & Njardarson, J. T. (2014). Mild stereoselective formation of tri- and tetrasubstituted olefins by regioselective ring opening of 1,1-disubstituted vinyl oxiranes with dialkyl dithiophosphates. TETRAHEDRON LETTERS, 55(21), 3232-3234.More infoPresented in this Letter is a mild new method to stereoselectively ring open 1,1-disubstituted vinyl oxiranes using dialkyl dithiophosphate nucleophiles. This new reaction proceeds in toluene at room temperature to afford tri- and tetrasubstituted allylic alcohols with high Z-selectivity. The syn relationship between the alcohol and the newly incorporated dithiophosphate moiety presented an opportunity to evaluate the anionic hopping properties of the ring opening product. Treatment of the ring opening product with base results in a facile phosphate hopping followed by a selective thiolate 3-exo-trig instead of a 5-exo-tet cyclization. (C) 2014 Elsevier Ltd. All rights reserved.
- Ilardi, E. A., Vitaku, E., & Njardarson, J. T. (2014). Data-Mining for Sulfur and Fluorine: An Evaluation of Pharmaceuticals To Reveal Opportunities for Drug Design and Discovery. JOURNAL OF MEDICINAL CHEMISTRY, 57(7), 2832-2842.More infoAmong carbon, hydrogen, oxygen, and nitrogen, sulfur and fluorine are both leading constituents of the pharmaceuticals that comprise our medicinal history. In efforts to stimulate the minds of both the general public and expert scientist, statistics were collected from the trends associated with therapeutics spanning 12 disease categories (a total of 1969 drugs) from our new graphical montage compilation: disease focused pharmaceuticals posters. Each poster is a vibrant display of a collection of pharmaceuticals (including structural image, Food and Drug Administration (FDA) approval date, international nonproprietary name (INN), initial market name, and a color-coded subclass of function) organized chronologically and classified according to an association with a particular clinical indication. Specifically, the evolution and structural diversity of sulfur and the popular integration of fluorine into drugs introduced over the past 50 years are evaluated. The presented qualitative conclusions in this article aim to promote innovative insights into drug development.
- Smith, B. R., Eastman, C. M., & Njardarson, J. T. (2014). Beyond C, H, O, and N! Analysis of the Elemental Composition of U.S. FDA Approved Drug Architectures Miniperspective. JOURNAL OF MEDICINAL CHEMISTRY, 57(23), 9764-9773.More infoThe diversity of elements among U.S. Food and Drug Administration (FDA) approved pharmaceuticals is analyzed and reported, with a focus on atoms other than carbon, hydrogen, oxygen, and nitrogen. Our analysis reveals that sulfur, chlorine, fluorine, and phosphorous represent about 90% of elemental substitutions, with sulfur being the fifth most used element followed closely by chlorine, then fluorine and finally phosphorous in the eighth place. The remaining 10% of substitutions are represented by 16 other elements of which bromine, iodine, and iron occur most frequently. The most detailed parts of our analysis are focused on chlorinated drugs as a function of approval date, disease condition, chlorine attachment, and structure. To better aid our chlorine drug analyses, a new poster showcasing the structures of chlorinated pharmaceuticals was created specifically for this study. Phosphorus, bromine, and iodine containing drugs are analyzed closely as well, followed by a discussion about other elements.
- Smith, D. T., & Njardarson, J. T. (2014). A Scalable Rhodium-Catalyzed Intermolecular Aziridination Reaction. ANGEWANDTE CHEMIE-INTERNATIONAL EDITION, 53(17), 4278-4280.
- Vitaku, E., Smith, D. T., & Njardarson, J. T. (2014). Analysis of the Structural Diversity, Substitution Patterns, and Frequency of Nitrogen Heterocycles among US FDA Approved Pharmaceuticals. JOURNAL OF MEDICINAL CHEMISTRY, 57(24), 10257-10274.More infoNitrogen heterocycles are among the most significant structural components of pharmaceuticals. Analysis of our database of U.S. FDA approved drugs reveals that 59% of unique small-molecule drugs contain a nitrogen heterocycle. In this review we report on the top 25 most commonly utilized nitrogen heterocycles found in pharmaceuticals. The main part of our analysis is divided into seven sections: (1) three- and four-membered heterocycles, (2) five-, (3) six-, and (4) seven- and eight-membered heterocycles, as well as (5) fused, (6) bridged bicyclic, and (7) macrocyclic nitrogen heterocycles. Each section reveals the top nitrogen heterocyclic structures and their relative impact for that ring type. For the most commonly used nitrogen heterocycles, we report detailed substitution patterns, highlight common architectural cores, and discuss unusual or rare structures.
- Yang, Q., Draghici, C., Njardarson, J. T., Li, F., Smith, B. R., & Das, P. (2014). Evolution of an oxidative dearomatization enabled total synthesis of vinigrol. ORGANIC & BIOMOLECULAR CHEMISTRY, 12(2), 330-344.More infoThe evolution of the synthetic strategy resulting in a total synthesis of vinigrol is presented. Oxidative dearomatization/intramolecular Diels-Alder cycloaddition has served as the successful cornerstone for all of the approaches. Extensive radical cyclization efforts to form the tetracyclic core resulted in interesting and surprising reaction outcomes, none of which could be advanced to vinigrol. These cyclization obstacles were successfully overcome by using Heck instead of radical cyclizations. The total synthesis features a trifluoroethyl ether protecting group being used for the first time in organic synthesis. The logic of its selection and the group's importance beyond protecting the C8a hydroxyl group is presented along with a discussion of strategies for its removal. Because of the compact tetracyclic cage the route is built around many unusual reaction observations and solutions have emerged. For example, a first of its kind Grob fragmentation reaction featuring a trifluoroethyl leaving group has been uncovered, interesting interrupted selenium dioxide allylic oxidations have been observed as well as intriguing catalyst and counterion dependent directed hydrogenations.
- Njardarson, J. T. (2013). Catalytic Ring Expansion Adventures. Synlett, 787-803. doi:10.1055/s-0032-1318326More infoThis account summarizes the Njardarson group ring expansion journey from early vinyl oxirane explorations to the latest vinyl oxetane ring expansion discoveries. The evolution of the program and incredible success of Cu(hfacac)2 as catalyst is detailed. Application of these new ring expansion reactions to natural product, pharmaceutical and commodity chemical targets is discussed as well as our intriguing mechanistic journey and interesting entry into the world of chiral counterion catalysis.
- Njardarson, J. T., & Guo, B. (2013). Z-Selective Ring Opening of Vinyl Oxetanes with Dialkyl Dithiophosphate Nucleophiles. Chemical Communications, 49, 10802-10804. doi:10.1039/C3CC46660DMore infoDialkyl dithiophosphates selectively ring open vinyl oxetanes in excellent yields under mild reaction conditions to form useful allylic thiophosphate products with high Z-selectivity.
- Njardarson, J. T., & Ilardi, E. A. (2013). Ring Expansions of Vinyloxiranes, -thiiranes, and -aziridines: Synthetic Approaches, Challenges, and Catalytic Success Stories. Journal of Organic Chemistry, 78, 9533–9540.More infoRing expansion reactions of strained vinylic heterocyclic substrates have attracted the attention of the synthetic community for decades. Strategic manipulations of these organic architectures enable access to many useful synthetic intermediates. This paper highlights various methods for the ring expansion of vinyloxiranes, -thiiranes, and -aziridines described in the literature from 1964 to 2013.
- Njardarson, J. T., & Mack, D. J. (2013). Recent Advances in the Metal Catalyzed Ring Expansions of Three and Four Membered Rings. ACS Catalysis, 3, 272–286. doi:10.1021/cs300771dMore infoNew catalytic ring-expansion reactions of strained ring (hetero- and carbocyclic) substrates reported in the last six years (2006–2012) are presented. As evident from the diversity of new approaches, this is a vibrant area of research. Metals ranging from magnesium to gold have been used as catalysts. Some of these reactions allow access to enantioenriched products by employing catalysts decorated with chiral organic motifs (primarily C-2 symmetric).
- Njardarson, J. T., & Mack, D. J. (2013). Syntheses and Structural Confirmations of Members of a Heterocycle Containing Family of Labdane Diterpenoids. Angewandte Chemie International Edition, 52, 1543–1547. doi:10.1002/anie.201208412More infoBuilding with berries! Several labdane natural products have been synthesized for the first time by the combination of a copper-catalyzed vinyl oxirane ring expansion reaction with an abundant, inexpensive, chiral natural source (juniper berries; see scheme). These expedient (1–5 step) syntheses have resulted in the structural confirmations of five natural products and one reassignment. Reagent-controlled oxidation and 1,3-diene isomerization results are also presented.
- Njardarson, J. T., & Yang, Q. (2013). Base Mediated Deprotection Strategies for Trifluoroethyl Ether (TFEE), a New Alcohol Protecting Group. Tetrahedron letters, 54, 7080-7082.More infoA trifluoroethyl (TFE) ether is specifically introduced as a protecting group in organic chemistry. Its first strategic application and removal in the total synthesis of vinigrol is discussed. Two lithium base mediated deprotection strategies for its removal are presented in this Letter. In one deprotection approach, the trifluoroethyl ether is converted to a difluorovinyl ether and then catalytically cleaved using osmium tetraoxide, while in the second approach a difluorovinyl anion is formed and trapped with an electrophilic oxygen reagent (MoOPH) to form a labile difluoroacetate. To further aid the reader, a summary of approaches for forming trifluoroethyl ethers is included as well as a discussion of alternate deprotection strategies.
- Njardarson, J. T., Ilardi, E. A., & Vitaku, E. (2013). An In-Pharm-ative Educational Poster Anthology Highlighting the Therapeutic Agents That Chronicle Our Medicinal History. Journal of Chemical Education, 90, 1403–1405. doi:10.1021/ed4002317More infoDisease Focused Posters is a new, free, and readily accessible collection of graphical timelines that arrange the vast number of FDA approved pharmaceuticals according to a specific theme. Capitalizing on the elegance of structural imagery and the innovative functions of organic compounds, each poster (in its simplistic appearance) has the capacity to communicate a wealth of information that appeals to the educational desires of the public, from the non-scientist to the expert.
- Njardarson, J. T., Mack, D. J., Mustart, T. J., & Cheong, P. H. (2013). Mechanism and the Origins of Stereospecificity in Copper Catalyzed Ring Expansion of Vinyl Oxiranes: A Traceless Dual Transition Metal-Mediated Process. Journal of the American Chemical Society, 135, 1471–1475. doi:10.1021/ja310065zMore infoDensity functional theory computations of the Cu-catalyzed ring expansion of vinyloxiranes is mediated by a traceless dual Cu(I)-catalyst mechanism. Overall, the reaction involves a monomeric Cu(I)-catalyst, but a single key step, the Cu migration, requires two Cu(I)-catalysts for the transformation. This dual-Cu step is found to be a true double Cu(I) transition state rather than a single Cu(I) transition state in the presence of an adventitious, spectator Cu(I). Both Cu(I) catalysts are involved in the bond forming and breaking process. The single Cu(I) transition state is not a stationary point on the potential energy surface. Interestingly, the reductive elimination is rate-determining for the major diastereomeric product, while the Cu(I) migration step is rate-determining for the minor. Thus, while the reaction requires dual Cu(I) activation to proceed, kinetically, the presence of the dual-Cu(I) step is untraceable. The diastereospecificity of this reaction is controlled by the Cu migration step. Suprafacial migration is favored over antarafacial migration due to the distorted Cu π-allyl in the latter.
- Njardarson, J. T., Yang, Q., Draghici, C., & Li, F. (2013). Total Synthesis of Vinigrol. Angewandte Chemie International Edition, 52, 8648–8651. doi:10.1002/anie.201304624More infoCarbocyclic cage fight: The substrate-controlled total synthesis of vinigrol features a strategic oxidative dearomatization/Diels–Alder cycloaddition reaction and a subsequent palladium-catalyzed cyclization cascade to construct the carbocyclic core. The C4, C9, and C12 stereocenters were installed using either reduction or oxidation reactions, and the diterpenoid core was unraveled by a ring fragmentation reaction.