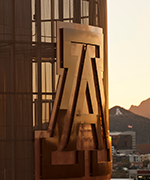
Pascale G Charest
- Associate Professor
- Associate Professor, Chemistry and Biochemistry-Sci
- Associate Professor, BIO5 Institute
- Member of the Graduate Faculty
- Associate Professor, Cancer Biology - GIDP
- (520) 626-2916
- Life Sciences South, Rm. 431
- Tucson, AZ 85721
- pcharest@arizona.edu
Biography
My group’s research focuses on the signal transduction pathways and molecular mechanisms controlling directed cell migration, or chemotaxis, in eukaryotic cells. Chemotaxis is central to many biological processes, including the embryonic development, wound healing, the migration of white blood cells (leukocytes) to sites of inflammation or bacterial infection, as well as the metastasis of cancer cells. Cells can sense chemical gradients that are as shallow as a 2% difference in concentration across the cell, and migrate towards the source of the signal, the chemoattractant. This is achieved through an intricate network of intracellular signaling pathways that are triggered by the chemoattractant signal. These pathways ultimately translate the detected chemoattractant gradient into changes in the cytoskeleton that lead to cell polarization and forward movement. In addition, many cells such as leukocytes and Dictyostelium, transmit the chemoattractant signal to other cells by themselves secreting chemoattractants, which increases the number of cells reaching the chemoattractant source.
To investigate the mechanisms of signal transduction underlying directed cell migration, we are using Dictyostelium discoideum, a model genetic system, as well as human cancer cell models. Cell motility and chemotaxis of Dictyostelium cells is very similar to that of leukocytes and cancer cells, using the same underlying cellular processes as these higher eukaryotic cells. We use Dictyostelium to identify proteins and mechanisms involved in chemotaxis and normal as well as malignant human cells to verify if our findings are conserved and implicated in cancer cell migration. We combine molecular genetic, proteomics and phosphoproteomics to identify new signaling proteins and pathways involved in the control of chemotaxis. We couple this approach with live cell imaging to understand the spatiotemporal dynamics of the signaling events, and with biochemical and biophysical analyses (including Bioluminescence Resonance Energy Transfer; BRET) to understand how proteins interact and function within the signaling networks.
Of note, in 2011 and in 2013, my career was disrupted due to the birth of two children, which affected my productivity at the early stages of my independent career. However, I have since successfully competed for major external funding and published several original research articles, demonstrating that my productivity and career is on an upward trajectory.
Degrees
- Ph.D. Biochemistry
- Universite de Montreal, Montreal, Quebec, Canada
- M.S. Biochemistry
- Universite de Montreal, Montreal, Quebec, Canada
- B.S. Biochemistry
- Universite de Sherbrooke, Sherbrooke, Quebec, Canada
Work Experience
- University of Arizona (2019 - Ongoing)
- University of Arizona, Tucson, Arizona (2012 - 2019)
- University of California, San Diego, La Jolla, California (2010 - 2012)
- University of California, San Diego, La Jolla, California (2005 - 2010)
- Universite de Montreal (2003 - 2005)
Awards
- MCB Faculty Innovator Award
- U. Arizona Dep. Molecular and Cellular Biology, Fall 2022
- Herbst Innovation Award
- College of Science, U. Arizona, Spring 2022
- Faculty Innovator Award
- Department of Molecular and Cellular Biology, U. Arizona, Fall 2019
- Camille Dreyfus Teacher-Scholar
- The Camille and Henry Dreyfus Foundation, Spring 2017 (Award Nominee)
- American Cancer Society Research Scholar
- American Cancer Society, Summer 2015
Interests
Teaching
Cellular Biology, Molecular Biology, Cellular Signaling, Cancer Biology, Biochemistry
Research
Cellular signaling, G protein-coupled receptors, Ras family GTPases, Target of Rapamycin Complex 2, Cell migration, Chemotaxis, Cancer metastasis
Courses
2025-26 Courses
-
Dissertation
BIOC 920 (Fall 2025) -
Dissertation
MCB 920 (Fall 2025) -
Exchange Chemical Info
CHEM 695B (Fall 2025) -
Lab Presentations+Discs
BIOC 696A (Fall 2025) -
Research
CHEM 900 (Fall 2025) -
Research
MCB 900 (Fall 2025) -
Special Tutoring Wkshp
MCB 497A (Fall 2025) -
The Biology of Cancer
MCB 325 (Fall 2025) -
Toolkit For The Rsrch Rookie
MCB 195C (Fall 2025)
2024-25 Courses
-
Honors Independent Study
MCB 299H (Summer I 2025) -
Internship
MCB 493 (Summer I 2025) -
Dissertation
BIOC 920 (Spring 2025) -
Dissertation
MCB 920 (Spring 2025) -
Exchange Chemical Info
CHEM 695B (Spring 2025) -
Honors Independent Study
MCB 399H (Spring 2025) -
Honors Thesis
BIOC 498H (Spring 2025) -
Internship
MCB 493 (Spring 2025) -
Research
BIOC 900 (Spring 2025) -
Thesis
BIOC 910 (Spring 2025) -
Directed Research
ABBS 792 (Fall 2024) -
Dissertation
BIOC 920 (Fall 2024) -
Dissertation
MCB 920 (Fall 2024) -
Honors Independent Study
MCB 299H (Fall 2024) -
Honors Independent Study
MCB 399H (Fall 2024) -
Honors Independent Study
MCB 499H (Fall 2024) -
Honors Thesis
BIOC 498H (Fall 2024) -
Lab Presentations+Discs
BIOC 696A (Fall 2024) -
Special Tutoring Wkshp
MCB 497A (Fall 2024) -
The Biology of Cancer
MCB 325 (Fall 2024)
2023-24 Courses
-
Internship
MCB 493 (Summer I 2024) -
Directed Rsrch
MCB 392 (Spring 2024) -
Dissertation
BIOC 920 (Spring 2024) -
Dissertation
MCB 920 (Spring 2024) -
Exchange Chemical Info
CHEM 695B (Spring 2024) -
Honors Directed Research
BIOC 492H (Spring 2024) -
Honors Independent Study
MCB 199H (Spring 2024) -
Honors Independent Study
MCB 399H (Spring 2024) -
Honors Independent Study
PCOL 299H (Spring 2024) -
Honors Thesis
BIOC 498H (Spring 2024) -
Honors Thesis
MCB 498H (Spring 2024) -
Lab Presentations & Discussion
MCB 696A (Spring 2024) -
Research
BIOC 900 (Spring 2024) -
Research
MCB 900 (Spring 2024) -
Directed Research
ABBS 792 (Fall 2023) -
Dissertation
BIOC 920 (Fall 2023) -
Dissertation
MCB 920 (Fall 2023) -
Exchange Chemical Info
CHEM 695B (Fall 2023) -
Honors Independent Study
MCB 399H (Fall 2023) -
Honors Independent Study
MCB 499H (Fall 2023) -
Honors Thesis
BIOC 498H (Fall 2023) -
Honors Thesis
MCB 498H (Fall 2023) -
Lab Presentations & Discussion
MCB 696A (Fall 2023) -
Research
MCB 900 (Fall 2023) -
Special Tutoring Wkshp
MCB 497A (Fall 2023) -
The Biology of Cancer
MCB 325 (Fall 2023)
2022-23 Courses
-
Directed Research
MCB 792 (Spring 2023) -
Directed Research
PSIO 492 (Spring 2023) -
Dissertation
BIOC 920 (Spring 2023) -
Dissertation
MCB 920 (Spring 2023) -
Exchange Chemical Info
CHEM 695B (Spring 2023) -
Honors Directed Research
BIOC 392H (Spring 2023) -
Honors Independent Study
MCB 399H (Spring 2023) -
Lab Presentations & Discussion
MCB 696A (Spring 2023) -
Lab Presentations+Discs
BIOC 696A (Spring 2023) -
MCB Journal Club
MCB 595 (Spring 2023) -
Seminar in Biochemistry
BIOC 696D (Spring 2023) -
Directed Research
MCB 792 (Fall 2022) -
Directed Rsrch
MCB 492 (Fall 2022) -
Dissertation
BIOC 920 (Fall 2022) -
Dissertation
MCB 920 (Fall 2022) -
Honors Directed Research
BIOC 392H (Fall 2022) -
Honors Independent Study
MCB 399H (Fall 2022) -
Lab Presentations & Discussion
MCB 696A (Fall 2022) -
Special Tutoring Wkshp
MCB 497A (Fall 2022) -
The Biology of Cancer
MCB 325 (Fall 2022)
2021-22 Courses
-
Directed Rsrch
MCB 392 (Summer I 2022) -
Directed Rsrch
MCB 492 (Summer I 2022) -
Directed Rsrch
MCB 492 (Spring 2022) -
Dissertation
BIOC 920 (Spring 2022) -
Dissertation
MCB 920 (Spring 2022) -
Exchange Chemical Info
CHEM 695B (Spring 2022) -
Honors Thesis
BIOC 498H (Spring 2022) -
Lab Presentations & Discussion
MCB 696A (Spring 2022) -
Research
BIOC 900 (Spring 2022) -
Directed Rsrch
MCB 392 (Fall 2021) -
Directed Rsrch
MCB 492 (Fall 2021) -
Dissertation
BIOC 920 (Fall 2021) -
Dissertation
MCB 920 (Fall 2021) -
Honors Thesis
BIOC 498H (Fall 2021) -
Lab Presentations & Discussion
MCB 696A (Fall 2021) -
Research
BIOC 900 (Fall 2021)
2020-21 Courses
-
Directed Rsrch
MCB 392 (Spring 2021) -
Directed Rsrch
MCB 492 (Spring 2021) -
Dissertation
BIOC 920 (Spring 2021) -
Exchange Chemical Info
CHEM 695B (Spring 2021) -
Honors Directed Research
BIOC 492H (Spring 2021) -
Honors Thesis
BIOC 498H (Spring 2021) -
Lab Presentations & Discussion
MCB 696A (Spring 2021) -
Research
MCB 900 (Spring 2021) -
Directed Research
ECOL 392 (Fall 2020) -
Directed Research
MCB 792 (Fall 2020) -
Directed Rsrch
MCB 392 (Fall 2020) -
Dissertation
CHEM 920 (Fall 2020) -
Exchange Chemical Info
CHEM 695B (Fall 2020) -
Honors Directed Research
BIOC 492H (Fall 2020) -
Honors Thesis
BIOC 498H (Fall 2020) -
Intro to Research in Biology
MCB 195C (Fall 2020) -
Lab Presentations & Discussion
MCB 696A (Fall 2020) -
Preceptorship
MCB 491 (Fall 2020) -
Research
MCB 900 (Fall 2020) -
The Biology of Cancer
MCB 325 (Fall 2020)
2019-20 Courses
-
Cell&Development Biology
MCB 305 (Spring 2020) -
Directed Research
MCB 792 (Spring 2020) -
Directed Rsrch
MCB 392 (Spring 2020) -
Dissertation
BIOC 920 (Spring 2020) -
Dissertation
CHEM 920 (Spring 2020) -
Exchange Chemical Info
CHEM 695B (Spring 2020) -
Honors Directed Research
BIOC 392H (Spring 2020) -
Honors Directed Research
BIOC 492H (Spring 2020) -
Independent Study
ECOL 399 (Spring 2020) -
Independent Study
MCB 399 (Spring 2020) -
Intro to Research in Biology
MCB 195C (Spring 2020) -
Research
BIOC 900 (Spring 2020) -
Science,Society + Ethics
CMM 695E (Spring 2020) -
Science,Society + Ethics
MCB 695E (Spring 2020) -
Senior Capstone
BIOC 498 (Spring 2020) -
Dissertation
CHEM 920 (Fall 2019) -
Exchange Chemical Info
CHEM 695B (Fall 2019) -
Honors Directed Research
BIOC 492H (Fall 2019) -
Honors Independent Study
BIOC 299H (Fall 2019) -
Introduction to Research
MCB 795A (Fall 2019) -
Research
CHEM 900 (Fall 2019) -
Senior Capstone
BIOC 498 (Fall 2019) -
What is MCB?
MCB 195I (Fall 2019)
2018-19 Courses
-
Directed Research
CHEM 392 (Spring 2019) -
Directed Rsrch
MCB 392 (Spring 2019) -
Dissertation
BIOC 920 (Spring 2019) -
Dissertation
CHEM 920 (Spring 2019) -
Exchange Chemical Info
CHEM 695B (Spring 2019) -
Honors Directed Research
BIOC 392H (Spring 2019) -
Honors Independent Study
CHEM 299H (Spring 2019) -
Honors Thesis
BIOC 498H (Spring 2019) -
Nucleic Acids, Metab & Signal
BIOC 568 (Spring 2019) -
Research
BIOC 900 (Spring 2019) -
Research
CHEM 900 (Spring 2019) -
Directed Research
BIOC 392 (Fall 2018) -
Dissertation
CHEM 920 (Fall 2018) -
Exchange Chemical Info
CHEM 695B (Fall 2018) -
Honors Directed Research
BIOC 392H (Fall 2018) -
Honors Independent Study
BIOC 199H (Fall 2018) -
Honors Thesis
BIOC 498H (Fall 2018) -
Intro to Biochem Rsrch
BIOC 296B (Fall 2018) -
Research
CHEM 900 (Fall 2018)
2017-18 Courses
-
Directed Research
BIOC 392 (Spring 2018) -
Dissertation
BIOC 920 (Spring 2018) -
Dissertation
CHEM 920 (Spring 2018) -
Exchange Chemical Info
CHEM 695B (Spring 2018) -
Honors Thesis
BIOC 498H (Spring 2018) -
Nucleic Acids, Metab & Signal
BIOC 568 (Spring 2018) -
Research
BIOC 900 (Spring 2018) -
Research
CHEM 900 (Spring 2018) -
Dissertation
CHEM 920 (Fall 2017) -
Exchange Chemical Info
CHEM 695B (Fall 2017) -
Honors Independent Study
BIOC 499H (Fall 2017) -
Honors Thesis
BIOC 498H (Fall 2017) -
Introduction to Research
BIOC 795A (Fall 2017) -
Introduction to Research
MCB 795A (Fall 2017) -
Research
CHEM 900 (Fall 2017)
2016-17 Courses
-
Directed Research
BIOC 392 (Spring 2017) -
Dissertation
BIOC 920 (Spring 2017) -
Exchange Chemical Info
CHEM 695B (Spring 2017) -
Honors Independent Study
BIOC 499H (Spring 2017) -
Lab Presentations+Discs
BIOC 696A (Spring 2017) -
Nucleic Acids, Metab & Signal
BIOC 568 (Spring 2017) -
Nucleic Acids, Metab & Signal
GENE 568 (Spring 2017) -
Research
BIOC 900 (Spring 2017) -
Senior Capstone
BIOC 498 (Spring 2017) -
Directed Research
BIOC 492 (Fall 2016) -
Dissertation
CHEM 920 (Fall 2016) -
Exchange Chemical Info
CHEM 695B (Fall 2016) -
Intro to Biochem Rsrch
BIOC 296B (Fall 2016) -
Introduction to Research
MCB 795A (Fall 2016) -
Research
CHEM 900 (Fall 2016) -
Senior Capstone
BIOC 498 (Fall 2016)
2015-16 Courses
-
Directed Research
BIOC 492 (Spring 2016) -
Dissertation
BIOC 920 (Spring 2016) -
Exchange Chemical Info
CHEM 695B (Spring 2016) -
Internship in Applied Biosci
ABS 593A (Spring 2016) -
Introduction to Research
MCB 795A (Spring 2016) -
Master's Report
ABS 909 (Spring 2016) -
Nucleic Acids, Metab & Signal
BIOC 568 (Spring 2016) -
Research
BIOC 900 (Spring 2016)
Scholarly Contributions
Journals/Publications
- Collins, S. E., Wiegand, M. E., Werner, A. N., Brown, I. N., Mundo, M. I., Swango, D. J., Mouneimne, G., & Charest, P. G. (2022). Ras-mediated activation of mTORC2 promotes breast epithelial cell migration and invasion. Molecular Biology of the Cell.More infoWe previously identified the mechanistic target of rapamycin complex 2 (mTORC2) as an effector of Ras for the control of directed cell migration in . Recently, the Ras-mediated regulation of mTORC2 was found to be conserved in mammalian cells, and mTORC2 was shown to be an effector of oncogenic Ras. Interestingly, mTORC2 has been linked to cancer cell migration, and particularly in breast cancer. Here, we investigated the role of Ras in promoting the migration and invasion of breast cancer cells through mTORC2. We observed that both Ras and mTORC2 promote the migration of different breast cancer cells and breast cancer cell models. Using HER2 and oncogenic Ras-transformed breast epithelial MCF10A cells, we found that both wild-type Ras and oncogenic Ras promote mTORC2 activation and an mTORC2-dependent migration and invasion in these breast cancer models. We further observed that, whereas oncogenic Ras-transformed MCF10A cells display uncontrolled cell proliferation and invasion, disruption of mTORC2 leads to loss of invasiveness only. Together, our findings suggest that, whereas the Ras-mediated activation of mTORC2 is expected to play a minor role in breast tumor formation, the Ras-mTORC2 pathway plays an important role in promoting the migration and invasion of breast cancer cells.
- Takashima, J. A., Woroniecka, H. A., & Charest, P. G. (2022). Proximity protein labeling in Dictyostelium with engineered ascorbic acid peroxidase 2.. Journal of Biological Methods.
- Smith, S. F., Collins, S. E., & Charest, P. G. (2020). Ras, PI3K and mTORC2 - three's a crowd?. Journal of cell science, 133(19).More infoThe Ras oncogene is notoriously difficult to target with specific therapeutics. Consequently, there is interest to better understand the Ras signaling pathways to identify potential targetable effectors. Recently, the mechanistic target of rapamycin complex 2 (mTORC2) was identified as an evolutionarily conserved Ras effector. mTORC2 regulates essential cellular processes, including metabolism, survival, growth, proliferation and migration. Moreover, increasing evidence implicate mTORC2 in oncogenesis. Little is known about the regulation of mTORC2 activity, but proposed mechanisms include a role for phosphatidylinositol (3,4,5)-trisphosphate - which is produced by class I phosphatidylinositol 3-kinases (PI3Ks), well-characterized Ras effectors. Therefore, the relationship between Ras, PI3K and mTORC2, in both normal physiology and cancer is unclear; moreover, seemingly conflicting observations have been reported. Here, we review the evidence on potential links between Ras, PI3K and mTORC2. Interestingly, data suggest that Ras and PI3K are both direct regulators of mTORC2 but that they act on distinct pools of mTORC2: Ras activates mTORC2 at the plasma membrane, whereas PI3K activates mTORC2 at intracellular compartments. Consequently, we propose a model to explain how Ras and PI3K can differentially regulate mTORC2, and highlight the diversity in the mechanisms of mTORC2 regulation, which appear to be determined by the stimulus, cell type, and the molecularly and spatially distinct mTORC2 pools.
- Islam, T. A., Scavello, M., Lotfi, P., Haldeman, P., Daniel, D., & Charest, P. G. (2019). Caffeine inhibits PI3K and mTORC2 in Dictyostelium and differentially affects multiple other cAMP chemoattractant signaling effectors. Molecular and Cellular Biochemistry.
- Islam, T. A., Yue, H., Scavello, M., Haldeman, P., Rappel, W. J., & Charest, P. G. (2018). The cAMP-induced G protein subunits dissociation monitored in live Dictyostelium cells by BRET reveals two activation rates, a positive effect of caffeine and potential role of microtubules. Cellular Signaling.
- Scavello, M., Petlick, A. R., Thompson, V. F., Ramesh, R., Lotfi, P., & Charest, P. G. (2017). Protein Kinase A spatiotemporally controls chemoattractant signaling pathways and is critical for gradient sensing in Dictyostelium. Journal of Cell Science. doi:10.1242/jcs.177170
- Islam, T. A., Stepanski, B. M., & Charest, P. G. (2016). Studying chemoattractant signal transduction dynamics in Dictyostelium using BRET. Methods in Molecular Biology, 1407, 63-77. doi:10.1007/978-1-4939-3480-5_5
- Khanna, A., Lotfi, P., Chavan, A. J., Montano, N. M., Bolourani, P., Weeks, G., Shen, Z., Briggs, S. P., Pots, H., Van Haastert, P. J., Kortholt, A., & Charest, P. G. (2016). The small GTPases Ras and Rap1 bind to and control TORC2 activity. Scientific Reports, 6, 25823. doi:10.1038/srep25823
- Sumita, K., Yoshino, H., Sasaki, M., Majd, N., Kahoud, E. R., Takahashi, H., Takeuchi, K., Kuroda, T., Lee, S., Charest, P. G., Takeda, K., Asara, J. M., Firtel, R. A., Anastasiou, D., & Sasaki, A. T. (2014). Degradation of activated K-ras orthologue via K-ras-specific lysine residues is required for cytokinesis. Journal of Biological Chemistry, 289(7), 3950-3959.More infoAbstract: Background: Targeting oncogenic K-Ras for cancer therapy has remained challenging. Results: Ubiquitination specifically occurs on the activated K-Ras orthologue in Dictyostelium via evolutionary conserved K-Ras lysines, which promotes K-Ras protein degradation. Conclusion: Our results indicate the existence of GTP-loaded K-Ras orthologue-specific degradation system in Dictyostelium. Significance: This work reveals a novel negative feedback regulation for the K-Ras isoform, which is critical for cytokinesis in Dictyostelium. © 2014 by The American Society for Biochemistry and Molecular Biology, Inc..
- Kölsch, V., Shen, Z., Lee, S., Plak, K., Lotfi, P., Chang, J., Charest, P. G., Romero, J. L., Jeon, T. J., Kortholt, A., Briggs, S. P., & Firtel, R. A. (2013). Daydreamer, a Ras effector and GSK-3 substrate, is important for directional sensing and cell motility. Molecular Biology of the Cell, 24(2), 100-114.More infoPMID: 23135995;PMCID: PMC3541958;Abstract: How independent signaling pathways are integrated to holistically control a biological process is not well understood. We have identified Daydreamer (DydA), a new member of the Mig10/RIAM/lamellipodin (MRL) family of adaptor proteins that localizes to the leading edge of the cell. DydA is a putative Ras effector that is required for cell polarization and directional movement during chemotaxis. dydA- cells exhibit elevated F-actin and assembled myosin II (MyoII), increased and extended phosphoinositide-3-kinase (PI3K) activity, and extended phosphorylation of the activation loop of PKB and PKBR1, suggesting that DydA is involved in the negative regulation of these pathways. DydA is phosphorylated by glycogen synthase kinase-3 (GSK-3), which is required for some, but not all, of DydA's functions, including the proper regulation of PKB and PKBR1 and MyoII assembly. gskA- cells exhibit very strong chemotactic phenotypes, as previously described, but exhibit an increased rate of random motility. gskA- cells have a reduced MyoII response and a reduced level of phosphatidylinositol (3,4,5)-triphosphate production, but a highly extended recruitment of PI3K to the plasma membrane and highly extended kinetics of PKB and PKBR1 activation. Our results demonstrate that GSK-3 function is essential for chemotaxis, regulating multiple substrates, and that one of these effectors, DydA, plays a key function in the dynamic regulation of chemotaxis. © 2013 Kölsch et al.
- Takeda, K., Shao, D., Adler, M., Charest, P. G., Loomis, W. F., Levine, H., Groisman, A., Rappel, W., & Firtel, R. A. (2012). Cell biology: Incoherent feedforward control governs adaptation of activated ras in a eukaryotic chemotaxis pathway. Science Signaling, 5(205).More infoPMID: 22215733;PMCID: PMC3928814;Abstract: Adaptation in signaling systems, during which the output returns to a fixed baseline after a change in the input, often involves negative feedback loops and plays a crucial role in eukaryotic chemotaxis. We determined the dynamical response to a uniform change in chemoattractant concentration of a eukaryotic chemotaxis pathway immediately downstream from G protein - coupled receptors. The response of an activated Ras showed near-perfect adaptation, leading us to attempt to fit the results using mathematical models for the two possible simple network topologies that can provide perfect adaptation. Only the incoherent feedforward network accurately described the experimental results. This analysis revealed that adaptation in this Ras pathway is achieved through the proportional activation of upstream components and not through negative feedback loops. Furthermore, these results are consistent with a local excitation, global inhibition mechanism for gradient sensing, possibly with a Ras guanosine triphosphatase - activating protein acting as a global inhibitor.
- Hecht, I., Skoge, M. L., Charest, P. G., Ben-Jacob, E., Firtel, R. A., Loomis, W. F., Levine, H., & Rappel, W. (2011). Activated membrane patches guide chemotactic cell motility. PLoS Computational Biology, 7(6).More infoPMID: 21738453;PMCID: PMC3127810;Abstract: Many eukaryotic cells are able to crawl on surfaces and guide their motility based on environmental cues. These cues are interpreted by signaling systems which couple to cell mechanics; indeed membrane protrusions in crawling cells are often accompanied by activated membrane patches, which are localized areas of increased concentration of one or more signaling components. To determine how these patches are related to cell motion, we examine the spatial localization of RasGTP in chemotaxing Dictyostelium discoideum cells under conditions where the vertical extent of the cell was restricted. Quantitative analyses of the data reveal a high degree of spatial correlation between patches of activated Ras and membrane protrusions. Based on these findings, we formulate a model for amoeboid cell motion that consists of two coupled modules. The first module utilizes a recently developed two-component reaction diffusion model that generates transient and localized areas of elevated concentration of one of the components along the membrane. The activated patches determine the location of membrane protrusions (and overall cell motion) that are computed in the second module, which also takes into account the cortical tension and the availability of protrusion resources. We show that our model is able to produce realistic amoeboid-like motion and that our numerical results are consistent with experimentally observed pseudopod dynamics. Specifically, we show that the commonly observed splitting of pseudopods can result directly from the dynamics of the signaling patches. © 2011 Hecht et al.
- Charest, P. G., & Firtel, R. A. (2010). TORCing Neutrophil Chemotaxis. Developmental Cell, 19(6), 795-796.More infoPMID: 21145496;PMCID: PMC3033560;Abstract: During cell migration, chemoattractant-induced signaling pathways determine the direction of movement by controlling the spatiotemporal dynamics of cytoskeletal components. In this issue of Developmental Cell, Liu et al. report that the target of rapamycin complex 2 (TORC2) controls cell polarity and chemotaxis through regulation of both F-actin and myosin II in migrating neutrophils. © 2010 Elsevier Inc.
- Charest, P. G., Shen, Z., Lakoduk, A., Sasaki, A. T., Briggs, S. P., & Firtel, R. A. (2010). A ras signaling complex controls the RasC-TORC2 pathway and directed cell migration. Developmental Cell, 18(5), 737-749.More infoPMID: 20493808;PMCID: PMC2893887;Abstract: Ras was found to regulate Dictyostelium chemotaxis, but the mechanisms that spatially and temporally control Ras activity during chemotaxis remain largely unknown. We report the discovery of a Ras signaling complex that includes the Ras guanine exchange factor (RasGEF) Aimless, RasGEFH, protein phosphatase 2A (PP2A), and a scaffold designated Sca1. The Sca1/RasGEF/PP2A complex is recruited to the plasma membrane in a chemoattractant- and F-actin-dependent manner and is enriched at the leading edge of chemotaxing cells where it regulates F-actin dynamics and signal relay by controlling the activation of RasC and the downstream target of rapamycin complex 2 (TORC2)-Akt/protein kinase B (PKB) pathway. In addition, PKB and PKB-related PKBR1 phosphorylate Sca1 and regulate the membrane localization of the Sca1/RasGEF/PP2A complex, and thereby RasC activity, in a negative feedback fashion. Thus, our study uncovered a molecular mechanism whereby RasC activity and the spatiotemporal activation of TORC2 are tightly controlled at the leading edge of chemotaxing cells. © 2010 Elsevier Inc.
- Kölsch, V., Charest, P. G., & Firtel, R. A. (2008). The regulation of cell motility and chemotaxis by phospholipid signaling. Journal of Cell Science, 121(5), 551-559.More infoPMID: 18287584;PMCID: PMC2671295;Abstract: Phosphoinositide 3-kinase (P13K), PTEN and localized phosphatidylinositol (3,4,5)-trisphosphate [PtdIns(3,4,5)P3] play key roles in chemotaxis, regulating cell motility by controlling the actin cytoskeleton in Dictyostelium and mammalian cells. PtdIns(3,4,5)P3, produced by PI3K, acts via diverse downstream signaling components, including the GTPase Rac, Arf-GTPases and the kinase Akt (PKB). It has become increasingly apparent, however, that chemotaxis results from an interplay between the P13K-PTEN pathway and other parallel pathways in Dictyostelium and mammalian cells. In Dictyostelium, the phospholipase PLA2 acts in concert with P13K to regulate chemotaxis, whereas phospholipase C (PLC) plays a supporting role in modulating P13K activity. In adenocarcinoma cells, PLC and the actin regulator cofilin seem to provide the direction-sensing machinery, whereas P13K might regulate motility.
- Zhang, S., Charest, P. G., & Firtel, R. A. (2008). Spatiotemporal Regulation of Ras Activity Provides Directional Sensing. Current Biology, 18(20), 1587-1593.More infoPMID: 18948008;PMCID: PMC2590931;Abstract: Cells' ability to detect and orient themselves in chemoattractant gradients has been the subject of numerous studies, but the underlying molecular mechanisms remain largely unknown [1]. Ras activation is the earliest polarized response to chemoattractant gradients downstream from heterotrimeric G proteins in Dictyostelium, and inhibition of Ras signaling results in directional migration defects [2]. Activated Ras is enriched at the leading edge, promoting the localized activation of key chemotactic effectors, such as PI3K and TORC2 [2-5]. To investigate the role of Ras in directional sensing, we studied the effect of its misregulation by using cells with disrupted RasGAP activity. We identified an ortholog of mammalian NF1, DdNF1, as a major regulator of Ras activity in Dictyostelium. We show that disruption of nfaA leads to spatially and temporally unregulated Ras activity, causing cytokinesis and chemotaxis defects. By using unpolarized, latrunculin-treated cells, we show that tight regulation of Ras is important for gradient sensing. Together, our findings suggest that Ras is part of the cell's compass and that the RasGAP-mediated regulation of Ras activity affects directional sensing. © 2008 Elsevier Ltd. All rights reserved.
- Charest, P. G., & Firtel, R. A. (2007). Big roles for small GTPases in the control of directed cell movement. Biochemical Journal, 401(2), 377-390.More infoPMID: 17173542;PMCID: PMC1820805;Abstract: Small GTPases are involved in the control of diverse cellular behaviours, including cellular growth, differentiation and motility. In addition, recent studies have revealed new roles for small GTPases in the regulation of eukaryotic chemotaxis. Efficient chemotaxis results from co-ordinated chemoattractant gradient sensing, cell polarization and cellular motility, and accumulating data suggest that small GTPase signalling plays a central role in each of these processes as well as in signal relay. The present review summarizes these recent findings, which shed light on the molecular mechanisms by which small GTPases control directed cell migration. © 2007 Biochemical Society.
- Charest, P. G., Oligny-Longpré, G., Bonin, H., Azzi, M., & Bouvier, M. (2007). The V2 vasopressin receptor stimulates ERK1/2 activity independently of heterotrimeric G protein signalling. Cellular Signalling, 19(1), 32-41.More infoPMID: 16857342;Abstract: The V2 vasopressin receptor (V2R) activates the mitogen activated protein kinases (MAPK) ERK1/2 through a mechanism involving the scaffolding protein βarrestin. Here we report that this activating pathway is independent of Gαs, Gαi, Gαq or Gβγ and that the V2R-mediated activation of Gαs inhibits ERK1/2 activity in a cAMP/PKA-dependent manner. In the HEK293 cells studied, the βarrestin-promoted activation was found to dominate over the PKA-mediated inhibition of the pathway, leading to a strong vasopressin-stimulated ERK1/2 activation. Despite the strong MAPK activation and in contrast with other GPCR, V2R did not induce any significant increase in DNA synthesis, consistent with the notion that the stable interaction between V2R and βarrestin prevents signal propagation to the nucleus. βarrestin was found to be essential for the ERK1/2 activation, indicating that the recruitment of the scaffolding protein is necessary and sufficient to initiate the signal in the absence of any other stimulatory cues. Based on the use of selective pharmacological inhibitors, dominant negative mutants and siRNA, we conclude that the βarrestin-dependent activation of ERK1/2 by the V2R involves c-Src and a metalloproteinase-dependent trans-activation event. These findings demonstrate that βarrestin is a genuine signalling initiator that can, on its own, engage a MAPK activation machinery upon stimulation of a GPCR by its natural ligand. © 2006 Elsevier Inc. All rights reserved.
- Hamdan, F. F., Rochdi, M. D., Breton, B., Fessart, D., Michaud, D. E., Charest, P. G., Laporte, S. A., & Bouvier, M. (2007). Unraveling G protein-coupled receptor endocytosis pathways using real-time monitoring of agonist-promoted interaction between β-arrestins and AP-2. Journal of Biological Chemistry, 282(40), 29089-29100.More infoPMID: 17675294;Abstract: The most widely studied pathway underlying agonist-promoted internalization of G protein-coupled receptors (GPCRs) involves β-arrestin and clathrin-coated pits. However, both β-arrestin- and clathrin-independent processes have also been reported. Classically, the endocytic routes are characterized using pharmacological inhibitors and various dominant negative mutants, resulting sometimes in conflicting results and interpretational difficulties. Here, taking advantage of the fact that β-arrestin binding to the β2 subunit of the clathrin adaptor AP-2 (β2-adaptin) is needed for the β-arrestin-mediated targeting of GPCRs to clathrin-coated pits, we developed a bioluminescence resonance energy transfer-based approach directly assessing the molecular steps involved in the endocytosis of GPCRs in living cells. For 10 of the 12 receptors tested, including some that were previously suggested to internalize via clathrin-independent pathways, agonist stimulation promoted β-arrestin 1 and 2 interaction with β2-adaptin, indicating a β-arrestin-and clathrin-dependent endocytic process. Detailed analyses of β-arrestin interactions with both the receptor and β2-adaptin also allowed us to demonstrate that recruitment of β-arrestins to the receptor and the ensuing conformational changes are the leading events preceding AP-2 engagement and subsequent clathrin-mediated endocytosis. Among the receptors tested, only the endothelin A and B receptors failed to promote interaction between β-arrestins and β2-adaptin. However, both receptors recruited β-arrestins upon agonist stimulation, suggesting a β-arrestin- dependent but clathrin-independent route of internalization for these two receptors. In addition to providing a new tool to dissect the molecular events involved in GPCR endocytosis, the bioluminescence resonance energy transfer-based β-arrestin/β2-adaptin interaction assay represents a novel biosensor to assess receptor activation. © 2007 by The American Society for Biochemistry and Molecular Biology, Inc.
- Sasaki, A. T., Janetopoulos, C., Lee, S., Charest, P. G., Takeda, K., Sundheimer, L. W., Meili, R., Devreotes, P. N., & Firtel, R. A. (2007). G protein-independent Ras/PI3K/F-actin circuit regulates basic cell motility. Journal of Cell Biology, 178(2), 185-191.More infoPMID: 17635933;PMCID: PMC2064438;Abstract: Phosphoinositide 3-kinase (PI3K)γ and Dictyostelium PI3K are activated via G protein-coupled receptors through binding to the Gβγ subunit and Ras. However, the mechanistic role(s) of Gβγ and Ras in PI3K activation remains elusive. Furthermore, the dynamics and function of PI3K activation in the absence of extracellular stimuli have not been fully investigated. We report that gβ null cells display PI3K and Ras activation, as well as the reciprocal localization of PI3K and PTEN, which lead to local accumulation of PI(3,4,5)P3. Simultaneous imaging analysis reveals that in the absence of extracellular stimuli, autonomous PI3K and Ras activation occur, concurrently, at the same sites where F-actin projection emerges. The loss of PI3K binding to Ras - guanosine triphosphate abolishes this PI3K activation, whereas prevention of PI3K activity suppresses autonomous Ras activation, suggesting that PI3K and Ras form a positive feedback circuit. This circuit is associated with both random cell migration and cytokinesis and may have initially evolved to control stochastic changes in the cytoskeleton. © The Rockefeller University Press.
- Charest, P. G., & Firtel, R. A. (2006). Feedback signaling controls leading-edge formation during chemotaxis. Current Opinion in Genetics and Development, 16(4), 339-347.More infoPMID: 16806895;Abstract: Chemotactic cells translate shallow chemoattractant gradients into a highly polarized intracellular response that includes the localized production of PI(3,4,5)P3 on the side of the cell facing the highest chemoattractant concentration. Research over the past decade began to uncover the molecular mechanisms involved in this localized signal amplification controlling the leading edge of chemotaxing cells. These mechanisms have been shown to involve multiple positive feedback loops, in which the PI(3,4,5)P3 signal amplifies itself independently of the original stimulus, as well as inhibitory signals that restrict PI(3,4,5)P3 to the leading edge, thereby creating a steep intracellular PI(3,4,5)P3 gradient. Molecules involved in positive feedback signaling at the leading edge include the small G-proteins Rac and Ras, phosphatidylinositol-3 kinase and F-actin, as part of interlinked feedback loops that lead to a robust production of PI(3,4,5)P3. © 2006 Elsevier Ltd. All rights reserved.
- Charest, P. G., Terrillon, S., & Bouvier, M. (2005). Monitoring agonist-promoted conformational changes of β-arrestin in living cells by intramolecular BRET. EMBO Reports, 6(4), 334-340.More infoPMID: 15776020;PMCID: PMC1299283;Abstract: Recruitment of β-arrestin (β-arr) to agonist-stimulated G-protein-coupled receptors (GPCRs) has a crucial role in controlling signalling efficacy and selectivity. When translocated to the receptor, β-arr is believed to undergo important conformational rearrangement necessary for its downstream actions. To probe these changes in living cells, we constructed an intramolecular bioluminescence resonance energy transfer (BRET)-based biosensor, in which β-arr is sandwiched between the Renilla luciferase (Luc) and the yellow fluorescent protein (YFP). We show that the intramolecular BRET between Luc and YFP was significantly increased following GPCR activation, suggesting a conformational rearrangement bringing the amino terminus and carboxyl terminus of β-arr in closer proximity. Kinetic analysis showed that this conformational change follows the initial β-arr/receptor engagement. In addition to providing new insights into the agonist-induced conformational rearrangements of β-arr in living cells, the double-brilliance β-arr offers a universal biosensor for GPCR activation, allowing the study of native receptors in large-scale screening analysis. ©2005 European Molecular Biology Organization.
- Perroy, J., Pontier, S., Charest, P. G., Aubry, M., & Bouvier, M. (2004). Real-time monitoring of ubiquitination in living cells by BRET.. Nat Methods, 1(3), 203-208.More infoPMID: 15782195;Abstract: Ubiquitin has emerged as an important regulator of protein stability and function in organisms ranging from yeast to mammals. The ability to detect in situ changes in protein ubiquitination without perturbing the physiological environment of cells would be a major step forward in understanding the ubiquitination process and its consequences. Here, we describe a new method to study this dynamic post-translational modification in intact human embryonic kidney cells. Using bioluminescence resonance energy transfer (BRET), we measured the ubiquitination of beta-arrestin 2, a regulatory protein implicated in the modulation of G protein-coupled receptors. In addition to allowing the detection of basal and GPCR-regulated ubiquitination of beta-arrestin 2 in living cells, real-time BRET measurements permitted the recording of distinct ubiquitination kinetics that are dictated by the identity of the activated receptor. The ubiquitination BRET assay should prove to be a useful tool for studying the dynamic ubiquitination of proteins and for understanding which cellular functions are regulated by this post-translational event.
- Azzi, M., Charest, P. G., Angers, S., Rousseau, G., Kohout, T., Bouvier, M., & Piñeyro, G. (2003). β-arrestin-mediated activation of MAPK by inverse agonists reveals distinct active conformations for G protein-coupled receptors. Proceedings of the National Academy of Sciences of the United States of America, 100(20), 11406-11411.More infoPMID: 13679574;PMCID: PMC208770;Abstract: It is becoming increasingly clear that signaling via G protein-coupled receptors is a diverse phenomenon involving receptor interaction with a variety of signaling partners. Despite this diversity, receptor ligands are commonly classified only according to their ability to modify G protein-dependent signaling. Here we show that β2AR ligands like ICI118551 and propranolol, which are inverse agonists for Gs-stimulated adenylyl cyclase, induce partial agonist responses for the mitogen-activated protein kinases extracellular signal-regulated kinase (ERK) 1/2 thus behaving as dual efficacy ligands. ERK1/2 activation by dual efficacy ligands was not affected by ADP-ribosylation of Gαi and could be observed in S49-cyc- cells lacking Gαs indicating that, unlike the conventional agonist isoproterenol, these drugs induce ERK1/2 activation in a Gs/i-independent manner. In contrast, this activation was inhibited by a dominant negative mutant of β-arrestin and was abolished in mouse embryonic fibroblasts lacking β-arrestin 1 and 2. The role of β-arrestin was further confirmed by showing that transfection of β-arrestin 2 in these knockout cells restored ICI118551 promoted ERK1/2 activation. ICI118551 and propranolol also promoted β-arrestin recruitment to the receptor. Taken together, these observations suggest that β-arrestin recruitment is not an exclusive property of agonists, and that ligands classically classified as inverse agonists rely exclusively on β-arrestin for their positive signaling activity. This phenomenon is not unique to β2-adrenergic ligands because SR121463B, an inverse agonist on the V2 vasopressin receptor-stimulated adenylyl cyclase, recruited β-arrestin and stimulated ERK1/2. These results point to a multistate model of receptor activation in which ligand-specific conformations are capable of differentially activating distinct signaling partners.
- Charest, P. G., & Bouvier, M. (2003). Palmitoylation of the V2 Vasopressin Receptor Carboxyl Tail Enhances β-Arrestin Recruitment Leading to Efficient Receptor Endocytosis and ERK1/ 2 Activation. Journal of Biological Chemistry, 278(42), 41541-41551.More infoPMID: 12900404;Abstract: A large number of G protein-coupled receptors are palmitoylated on cysteine residues located in their carboxyl tail, but the general role of this post-translational modification remains poorly understood. Here we show that preventing palmitoylation of the V2 vasopressin receptor, by site-directed mutagenesis of cysteines 341 and 342, significantly delayed and decreased both agonist-promoted receptor endocytosis and mitogen-activated protein kinase activation. Pharmacological blockade of receptor endocytosis is without effect on the vasopressin-stimulated mitogen-activated protein kinase activity, excluding the possibility that the reduced kinase activation mediated by the palmitoylation-less mutant could result from altered receptor endocytosis. In contrast, two dominant negative mutants of β-arrestin which inhibit receptor endocytosis also attenuated vasopressin-stimulated mitogen-activated protein kinase activity, suggesting that the scaffolding protein, β-arrestin, represents the common link among receptor palmitoylation, endocytosis, and kinase activation. Co-immunoprecipitation and bioluminescence resonance energy transfer experiments confirmed that inhibiting receptor palmitoylation considerably reduced the vasopressin-stimulated recruitment of β-arrestin to the receptor. Interestingly, the changes in β-arrestin recruitment kinetics were similar to those observed for vasopressin-stimulated receptor endocytosis and mitogen-activated protein kinase activation. Taken together the results indicate that palmitoylation enhances the recruitment of β-arrestin to the activated V2 vasopressin receptor thus facilitating processes requiring the scaffolding action of β-arrestin.
Presentations
- Charest, P. G. (2022, April). Understanding a cell's sense of direction. Quantitative Biology seminar series. Department of Mathematics: U. Arizona.
- Charest, P. G. (2022, Feb). Ras, mTOR, and cancer cell migration. Cancer Biology seminar series, UACCU. Arizona.
- Charest, P. G. (2022, March). Ras proteins in cell migration. BECUR Conference - Keynote speaker. U. Arizona: U. Arizona.
- Charest, P. G. (2021, April). Ras, mTOR, and cancer cell migration. Collaborative Cancer Grand Rounds, UACCU. Arizona.
- Charest, P. G. (2019, May). Understanding chemical attraction... of cells. Joint Seminar seriesDepartment of Molecular and Cellular Biology, U. Arizona.
- Charest, P. G. (2019, Summer). The effect of caffeine on Dictyostelium. International Dictyostelium Conference. Ann Harbor, MI.
- Collins, S., Brown, I., & Charest, P. G. (2019, January). Mechanistic target of Rapamycin Complex 2 (mTORC2) regulation in cancer cell migration. Gordon Research Conference on Directed Cell Migration. Galveston, TX.
- Charest, P. G. (2018, December). Understanding chemical attraction of cells. Invited Seminar. Department of Biomedical Sciences, New York Institute of Technology, Old Westbury, NY.
- Charest, P. G. (2018, January). Signaling mechanisms controlling directed cell migration. Invited seminar. Department of Biology, Texas A&M University, College Station, TX.
- Charest, P. G. (2018, June). Quantitative analyses of chemoattractant-induced heterotrimeric G protein activation kinetics in Dictyostelium reveal important role for high affinity pre-coupled receptors. Gordon Research Conference on Phosphorylation and G Protein-Mediated Signaling Networks. Biddeford, ME.
- Charest, P. G. (2017, March). Signaling mechanisms controlling directed cell migration. Seminar, Microbiology and Immunobiology, University of ArizonaUniversity of Arizona.
- Charest, P. G. (2017, November). Signaling mechanisms undelrying directed cell migration. Seminar, Department of Biological Sciences, Hunter College. New York, NY: Hunter College, City University of New York.
- Charest, P. G. (2017, September). Signaling mechanisms undelrying directed cell migration. Seminar, Department of Genetics, Cell Biology and Development, University of Minnesota. Minneapolis, MN: University of Minnesota.
- Charest, P. G. (2017, September). Signaling mechanisms undelrying directed cell migration. Seminar, Department of Microbiology & Molecular Genetics, Oklahoma State University. Stillwater, OK: Oklahoma State University.
- Charest, P. G. (2015, January). PKA promotes adaptation of chemoattractant signal transduction pathways and is critical for gradient sensing. Gordon Research Conference on Directed Cell Migration.
- Charest, P. G. (2015, November). Signaling mechanisms controlling directed cell migration. Seminar, Department of Basic Medical Sciences, University of Arizona COM-Phoenix. College of Medicine, University of Arizona, Phoenix.
- Charest, P. G. (2015, September). Signaling mechanisms controlling directed cell migration. Seminar, NIH/NIAID. NIH/NIAID Twinbrook campus, Rockville, MD: NIH/NIAID.
- Charest, P. G. (2015, September). Signaling mechanisms controlling directed cell migration. Seminar, Pennsylvania Muscle Institute, University of Pennsylvania. School of Medicine, University of Pennsylvania: University of Pennsylvania.
- Charest, P. (2014, June). The small GTPase Rap1 binds to and activates TORC2. Gordon Research Conference on Phosphorylation and G Protein Mediated Signaling Networks. Biddeford, ME.More infoPoster talk
- Charest, P. G. (2014, April). Signaling networks controlling chemotaxis. Arizona Research Institute for Biomedical Imaging (ARIBI) spring workshop. Tucson, AZ.
- Charest, P. G. (2013, August). Tight regulation of TORC2 function in chemotaxis. Annual International Dictyostelium Conference. Asheville, NC.
- Charest, P. G. (2013, February). The signaling networks underlying directed cell migration. Seminar, Drug Discovery and Developmental Therapeutics, University of Arizona. College of Pharmacy, University of Arizona.
- Charest, P. G. (2013, March). Signaling networks controlling chemotaxis. Arizona Imaging and Microanalysis Society annual conference. Tucson, Arizona.
- Charest, P. G. (2013, October). The signaling networks underlying directed cell migration. Seminar, Department of Immunobiology, University of Arizona. Department of Immunobiology, University of Arizona.
Poster Presentations
- Collins, S. E., Wiegand, M. E., Werner, A. N., Brown, I. N., Mundo, I. M., Mouneimne, G., & Charest, P. G. (2023, January). Ras-mediated activation of mTORC2 drives breast cancer cell migration and invasion. Gordon Research Conference on Directed Cell Migration. Galveston, TX: Gordon Research Conferences.
- Cahigas, G., Feldmann, I., & Charest, P. G. (2022, April). Determining the role of Ras family GTPase Rap1 in regulating mTORC2 activity and function in cell migration. American Society for Biochemistry and Molecular Biology, and Experimental Biology 2022 annual conference. Philadelphia, PA: ASBMB.
- Orlinski, A., & Charest, P. G. (2022, December). Characterizing the interaction between mTORC2 component SIN1 and the small GTPase Rap1. American Society for Cell Biology 2022 annual conference. Washington, DC: ASCB.
- Werner, A. N., Kumar, A. I., & Charest, P. G. (2022, December). The role and regulation of mTORC2 in lung cancer cell migration. American Society for Cell Biology 2022 annual conference. Washington, DC: ASCB.
- Wiegand, M. E., Collins, S. E., Brown, I. N., Werner, A. N., Mundo, I. M., Mouneimne, G., & Charest, P. G. (2022, April). Ras-mediated activation of mTORC2 drives breast cancer cell migration and invasion. American Association for Cancer Research 2022 annual conference. New Orleans, LA: American Association for Cancer Research.
- Takashima, J., Islam, A. T., & Charest, P. G. (2020, August). APEX2-mediated proximity labeling in Dictyostelium discoideum. 2020 Beckman Symposium.
- Collins, S., Brown, I., & Charest, P. G. (2019, January). Mechanistic target of Rapamycin Complex 2 (mTORC2) regulation in cancer cell migration. Gordon Research Conference on Directed Cell Migration. Galveston, TX.
- Smith, S. F., Weiss, E., & Charest, P. G. (2019, August). Investigating the Ras-mediated activation of mTORC2. International Dictyostelium Conference. Ann Harbor, MI.
- Takashima, J., Islam, A. T., Schultz, M., & Charest, P. G. (2019, August). Investigating the cAR1:Ga2bg interaction in Dictyostelium by Bioluminescence Resonance Energy Transfer. International Dictyostelium Conference.
- Charest, P. G. (2018, June). Quantitative analyses of chemoattractant-induced heterotrimeric G protein activation kinetics in Dictyostelium reveal important role for high affinity pre-coupled receptors. Gordon Research Conference on Phosphorylation and G Protein-Mediated Signaling Networks. Biddeford, ME.
- Charest, P. G. (2017, Jannuary). The role of PKA in Dictyostelium chemotaxis. Gordon Research Conference on Directed Cell Migration. Galveston, TX.
- Scavello, M., Petlick, A. R., Ramesh, R., Thompson, V. F., Lotfi, P., & Charest, P. G. (2017, January). The role of PKA in Dictyostelium chemotaxis. Gordon Conference on Directed Cell Migration. Galveston, TX.
- Islam, T. A., Haldeman, P., Stepanski, B. M., & Charest, P. G. (2016, August). Heterotrimeric G protein activation and regulation monitored by Bioluminescence Resonance Energy Transfer (BRET). Annual International Dictyostelium Conference.
- Khanna, A., Lotfi, P., Montano, N. M., Bolourani, P., Weeks, G., Shen, Z., Briggs, S. P., Pots, H., Van Haastert, P. J., Kortholt, A., & Charest, P. G. (2016, August). The small GTPases Ras and Rap1 bind to and control TORC2 activity. Annual International Dictyostelium Conference. Tucson, AZ.
- Islam, T. A., Stepanski, B. M., & Charest, P. G. (2015, December). Studying chemoattractant signal transduction dynamics in Dictyostelium by BRET. American Society for Cell Biology Annual Meeting.
- Khanna, A., Lotfi, P., Montano, N. M., Bolourani, P., Weeks, G., Shen, Z., Briggs, S. P., Pots, H., Van Haastert, P. J., Kortholt, A., & Charest, P. G. (2015, December). The small GTPases Ras and Rap1 bind to and control TORC2 activity. American Society for Cell Biology Annual Meeting. San Diego, CA.
- Scavello, M., Petlick, A. R., Thompson, V. F., Lotfi, P., & Charest, P. G. (2015, December). PKA spatiotemporally controls chemoattractant signaling pathways and is critical for gradient sensing in Dictyostelium. American Society for Cell Biology Annual Meeting. San Diego, CA.
- Scavello, M., Petlick, A. R., Thompson, V. F., Lotfi, P., & Charest, P. G. (2015, January). PKA spatiotemporally controls chemoattractant signaling pathways and is critical for gradient sensing in Dictyostelium. Gordon Research Conference on Directed Cell Migration. Galveston, TX.
- Khanna, A., Lotfi, P., Montano, N. M., Bolourani, P., Weeks, G., Shen, Z., Briggs, S. P., Pots, H., Van Haastert, P. J., Kortholt, A., & Charest, P. G. (2014, June). The small GTPases Ras and Rap1 bind to and control TORC2 activity. Gordon Research Conference on phosphorylaiton and G protein-mediated signaling networks. Biddeford, ME.