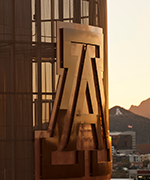
Ted Weinert
- Professor, Molecular and Cellular Biology
- Professor, Cancer Biology - GIDP
- Professor, Genetics - GIDP
- Member of the Graduate Faculty
Contact
- (520) 621-8511
- Life Sciences South, Rm. 000546
- Tucson, AZ 85721
- tweinert@arizona.edu
Biography
Not sure what you want here.
Degrees
- Ph.D. Molecular Genetics
- Yale University, New Haven, Connecticut, United States
- Bacterial Transposon IS903
Work Experience
- University of Arizona, Tucson, Arizona (1990 - Ongoing)
Awards
- Distinguished Career Teaching Award
- College of Science, Spring 2022
Licensure & Certification
- Professor, none, none (2015)
Interests
Research
Genome instability using budding yeast as a model
Teaching
bio181 and MCB572
Courses
2024-25 Courses
-
Cell Systems
MCB 572A (Fall 2024) -
MCB Seminar
MCB 596 (Fall 2024)
2023-24 Courses
-
Honors Thesis
ECOL 498H (Spring 2024) -
Honors Thesis
MCB 498H (Spring 2024) -
MCB Journal Club
MCB 595 (Spring 2024) -
MCB Seminar
MCB 596 (Spring 2024) -
Cell Systems
MCB 572A (Fall 2023) -
Honors Thesis
ECOL 498H (Fall 2023) -
Honors Thesis
MCB 498H (Fall 2023) -
MCB Journal Club
MCB 595 (Fall 2023) -
MCB Seminar
MCB 596 (Fall 2023) -
Scientific Communication
MCB 575 (Fall 2023)
2022-23 Courses
-
MCB Seminar
MCB 596 (Spring 2023) -
Cell Systems
MCB 572A (Fall 2022) -
Introductory Biology I
MCB 181R (Fall 2022) -
Preceptorship
MCB 391 (Fall 2022) -
Special Tutoring Wkshp
MCB 497A (Fall 2022)
2021-22 Courses
-
Biologist Approaches Big Probl
MCB 195J (Spring 2022) -
MCB Seminar
MCB 596 (Spring 2022) -
Cell Systems
MCB 572A (Fall 2021) -
Introductory Biology I
MCB 181R (Fall 2021)
2020-21 Courses
-
MCB Seminar
MCB 596 (Spring 2021) -
CBIO GIDP Seminar Series
CBIO 596H (Fall 2020) -
Cell Systems
MCB 572A (Fall 2020) -
Introductory Biology I
MCB 181R (Fall 2020) -
MCB Journal Club
MCB 595 (Fall 2020) -
MCB Seminar
MCB 596 (Fall 2020)
2019-20 Courses
-
Biologist Approaches Big Probl
MCB 195J (Spring 2020) -
CBIO GIDP Seminar Series
CBIO 596H (Fall 2019) -
Cell Systems
MCB 572A (Fall 2019) -
Introductory Biology I
MCB 181R (Fall 2019) -
MCB Seminar
MCB 596 (Fall 2019)
2018-19 Courses
-
CBIO GIDP Seminar Series
CBIO 596H (Fall 2018) -
Cell Systems
MCB 572A (Fall 2018) -
Introductory Biology I
MCB 181R (Fall 2018)
2017-18 Courses
-
Lab Present & Discuss
MCB 496A (Spring 2018) -
CBIO GIDP Seminar Series
CBIO 596H (Fall 2017) -
Cell Systems
MCB 572A (Fall 2017) -
Introductory Biology I
MCB 181R (Fall 2017) -
Topic Molec Biology
MCB 595A (Fall 2017)
2016-17 Courses
-
Honors Independent Study
MCB 499H (Spring 2017) -
Honors Independent Study
PSIO 499H (Spring 2017) -
Honors Thesis
MCB 498H (Spring 2017) -
CBIO GIDP Seminar Series
CBIO 596H (Fall 2016) -
Cell Systems
MCB 572A (Fall 2016) -
Dissertation
MCB 920 (Fall 2016) -
Honors Independent Study
MCB 499H (Fall 2016) -
Honors Independent Study
NSCS 399H (Fall 2016) -
Honors Thesis
MCB 498H (Fall 2016) -
Introductory Biology I
MCB 181R (Fall 2016) -
Lab Presentations & Discussion
MCB 696A (Fall 2016) -
Topic Molec Biology
MCB 595A (Fall 2016)
2015-16 Courses
-
CBIO GIDP Seminar Series
CBIO 596H (Spring 2016) -
Departmental Seminar
CMM 696A (Spring 2016) -
Dissertation
MCB 920 (Spring 2016) -
Honors Independent Study
MCB 499H (Spring 2016) -
Honors Thesis
MCB 498H (Spring 2016) -
Lab Presentations & Discussion
MCB 696A (Spring 2016) -
Topic Molec Biology
MCB 595A (Spring 2016)
Scholarly Contributions
Journals/Publications
- Langston, R. E., Palazzola, D., Bonnell, E., Wellinger, R. J., & Weinert, T. (2020). Loss of Cdc13 causes genome instability by a deficiency in replication-dependent telomere capping. PLoS genetics, 16(4), e1008733.More infoIn budding yeast, Cdc13, Stn1, and Ten1 form the telomere-binding heterotrimer CST complex. Here we investigate the role of Cdc13/CST in maintaining genome stability by using a Chr VII disome system that can generate recombinants, chromosome loss, and enigmatic unstable chromosomes. In cells expressing a temperature sensitive CDC13 allele, cdc13F684S, unstable chromosomes frequently arise from problems in or near a telomere. We found that, when Cdc13 is defective, passage through S phase causes Exo1-dependent ssDNA and unstable chromosomes that are then the source for additional chromosome instability events (e.g. recombinants, chromosome truncations, dicentrics, and/or chromosome loss). We observed that genome instability arises from a defect in Cdc13's function during DNA replication, not Cdc13's putative post-replication telomere capping function. The molecular nature of the initial unstable chromosomes formed by a Cdc13-defect involves ssDNA and does not involve homologous recombination nor non-homologous end joining; we speculate the original unstable chromosome may be a one-ended double strand break. This system defines a link between Cdc13's function during DNA replication and genome stability in the form of unstable chromosomes, that then progress to form other chromosome changes.
- Weinert, T., & Vinton, P. (2017). A Slowed Cell Cycle Stabilizes the Budding Yeast Genome. Genetics, 206, 811-828. doi:116.197590
- Langston, R. E., & Weinert, T. (2015). Nifty Alleles, a Plethora of Interactions, and Imagination Advance Understanding of Smc5/6's Roles with Chromosomes. Molecular cell, 60(6), 832-3.More infoThe Smc5/6 complex is involved in DNA replication and repair, but why it is essential is less clear. In this issue of Molecular Cell, Menolfi et al. (2015) use cell cycle-specific alleles to link the essential function of Smc5/6 to resolving replication problems that persist into G2.
- Beyer, T., & Weinert, T. (2014). Mec1 and Tel1: an arresting dance of resection. The EMBO journal.
- Carr, A. M., Paek, A. L., & Weinert, T. (2011). DNA replication: Failures and inverted fusions. Seminars in Cell and Developmental Biology, 22(8), 866-874.More infoPMID: 22020070;Abstract: DNA replication normally follows the rules passed down from Watson and Crick: the chromosome duplicates as dictated by its antiparallel strands, base-pairing and leading and lagging strand differences. Real-life replication is more complicated, fraught with perils posed by chromosome damage for one, and by transcription of genes and by other perils that disrupt progress of the DNA replication machinery. Understanding the replication fork, including DNA structures, associated replisome and its regulators, is key to understanding how cells overcome perils and minimize error. Replication fork error leads to genome rearrangements and, potentially, cell death. Interest in the replication fork and its errors has recently gained added interest by the results of deep sequencing studies of human genomes. Several pathologies are associated with sometimes-bizarre genome rearrangements suggestive of elaborate replication fork failures. To try and understand the links between the replication fork, its failure and genome rearrangements, we discuss here phases of fork behavior (stall, collapse, restart and fork failures leading to rearrangements) and analyze two examples of instability from our own studies; one in fission yeast and the other in budding yeast. © 2011.
- Weinert, T., Kaochar, S., Paek, A. L., & Weinert, T. -. (2010). Genetics. Replication error amplified. Science (New York, N.Y.), 329(5994).
- Weinert, T., Kaochar, S., Shanks, L., & Weinert, T. -. (2010). Checkpoint genes and Exo1 regulate nearby inverted repeat fusions that form dicentric chromosomes in Saccharomyces cerevisiae. Proceedings of the National Academy of Sciences of the United States of America, 107(50).More infoGenomic rearrangements are common, occur by largely unknown mechanisms, and can lead to human diseases. We previously demonstrated that some genome rearrangements occur in budding yeast through the fusion of two DNA sequences that contain limited sequence homology, lie in inverted orientation, and are within 5 kb of one another. This inverted repeat fusion reaction forms dicentric chromosomes, which are well-known intermediates to additional rearrangements. We have previously provided evidence indicating that an error of stalled or disrupted DNA replication forks can cause inverted repeat fusion. Here we analyze how checkpoint protein regulatory pathways known to stabilize stalled forks affect this form of instability. We find that two checkpoint pathways suppress inverted repeat fusion, and that their activities are distinguishable by their interactions with exonuclease 1 (Exo1). The checkpoint kinase Rad53 (Chk2) and recombination protein complex MRX(MRN) inhibit Exo1 in one pathway, whereas in a second pathway the ATR-like kinases Mec1 and Tel1, adaptor protein Rad9, and effector kinases Chk1 and Dun1 act independently of Exo1 to prevent inverted repeat fusion. We provide a model that indicates how in Rad53 or MRX mutants, an inappropriately active Exo1 may facilitate faulty template switching between nearby inverted repeats to form dicentric chromosomes. We further investigate the role of Rad53, using hypomorphic alleles of Rad53 and null mutations in Rad9 and Mrc1, and provide evidence that only local, as opposed to global, activity of Rad53 is sufficient to prevent inverted repeat fusion.
- Weinert, T., Paek, A. L., & Weinert, T. -. (2010). Choreography of the 9-1-1 checkpoint complex: DDK puts a check on the checkpoints. Molecular cell, 40(4).More infoCheckpoint proteins respond to DNA damage by halting the cell cycle until the damage is repaired. In this issue of Molecular Cell, Furuya et al. (2010) provide evidence that checkpoint proteins need to be removed from sites of damage in order to properly repair it.
- Weinert, T., Paek, A. L., Jones, H., Kaochar, S., & Weinert, T. -. (2010). The role of replication bypass pathways in dicentric chromosome formation in budding yeast. Genetics, 186(4).More infoGross chromosomal rearrangements (GCRs) are large scale changes to chromosome structure and can lead to human disease. We previously showed in Saccharomyces cerevisiae that nearby inverted repeat sequences (∼20-200 bp of homology, separated by ∼1-5 kb) frequently fuse to form unstable dicentric and acentric chromosomes. Here we analyzed inverted repeat fusion in mutants of three sets of genes. First, we show that genes in the error-free postreplication repair (PRR) pathway prevent fusion of inverted repeats, while genes in the translesion branch have no detectable role. Second, we found that siz1 mutants, which are defective for Srs2 recruitment to replication forks, and srs2 mutants had opposite effects on instability. This may reflect separate roles for Srs2 in different phases of the cell cycle. Third, we provide evidence for a faulty template switch model by studying mutants of DNA polymerases; defects in DNA pol delta (lagging strand polymerase) and Mgs1 (a pol delta interacting protein) lead to a defect in fusion events as well as allelic recombination. Pol delta and Mgs1 may collaborate either in strand annealing and/or DNA replication involved in fusion and allelic recombination events. Fourth, by studying genes implicated in suppression of GCRs in other studies, we found that inverted repeat fusion has a profile of genetic regulation distinct from these other major forms of GCR formation.
- Weinert, T., Kaochar, S., Jones, H., Paek, A., & Clark, A. (2009). The replication fork's five degrees of freedom, their failure and genome rearrangements. Current Opinion in Cell Biology, 21(6), 778-784.More infoPMID: 19913398;Abstract: Genome rearrangements are important in pathology and evolution. The thesis of this review is that the genome is in peril when replication forks stall, and stalled forks are normally rescued by error-free mechanisms. Failure of error-free mechanisms results in large-scale chromosome changes called gross chromosomal rearrangements, GCRs, by the aficionados. In this review we discuss five error-free mechanisms a replication fork may use to overcome blockage, mechanisms that are still poorly understood. We then speculate on how genome rearrangements may occur when such mechanisms fail. Replication fork recovery failure may be an important feature of the oncogenic process. (Feedback to the authors on topics discussed herein is welcome.).
- Weinert, T., Paek, A. L., Kaochar, S., Jones, H., Elezaby, A., Shanks, L., & Weinert, T. -. (2009). Fusion of nearby inverted repeats by a replication-based mechanism leads to formation of dicentric and acentric chromosomes that cause genome instability in budding yeast. Genes & development, 23(24).More infoLarge-scale changes (gross chromosomal rearrangements [GCRs]) are common in genomes, and are often associated with pathological disorders. We report here that a specific pair of nearby inverted repeats in budding yeast fuse to form a dicentric chromosome intermediate, which then rearranges to form a translocation and other GCRs. We next show that fusion of nearby inverted repeats is general; we found that many nearby inverted repeats that are present in the yeast genome also fuse, as does a pair of synthetically constructed inverted repeats. Fusion occurs between inverted repeats that are separated by several kilobases of DNA and share >20 base pairs of homology. Finally, we show that fusion of inverted repeats, surprisingly, does not require genes involved in double-strand break (DSB) repair or genes involved in other repeat recombination events. We therefore propose that fusion may occur by a DSB-independent, DNA replication-based mechanism (which we term "faulty template switching"). Fusion of nearby inverted repeats to form dicentrics may be a major cause of instability in yeast and in other organisms.
- Weinert, T., & Hopper, A. K. (2007). tRNA Traffic Meets a Cell-Cycle Checkpoint. Cell, 131(5), 838-840.More infoPMID: 18045528;Abstract: The molecular pathways linking DNA-damage checkpoint proteins to cell-cycle progression remain largely unresolved. Findings by Ghavidel et al. (2007) reported in this issue suggest that tRNA trafficking and the transcription factor Gcn4 are key intermediates in the process by which yeast cells detect DNA damage and delay cell-cycle progression at the G1 to S phase transition. © 2007 Elsevier Inc. All rights reserved.
- Weinert, T., & Weinert, T. -. (2007). Cell biology. What a cell should know (but may not). Science (New York, N.Y.), 315(5817).
- Admire, A., Shanks, L., Danzl, N., Wang, M., Weier, U., Stevens, W., Hunt, E., & Weinert, T. (2006). Cycles of chromosome instability are associated with a fragile site and are increased by defects in DNA replication and checkpoint controls in yeast. Genes and Development, 20(2), 159-173.More infoPMID: 16384935;PMCID: PMC1356108;Abstract: We report here that a normal budding yeast chromosome (ChrVII) can undergo remarkable cycles of chromosome instability. The events associated with cycles of instability caused a distinctive "sectoring" of colonies on selective agar plates. We found that instability initiated at any of several sites on ChrVII, and was sharply increased by the disruption of DNA replication or by defects in checkpoint controls. We studied in detail the cycles of instability associated with one particular chromosomal site (the "403 site"). This site contained multiple tRNA genes known to stall replication forks, and when deleted, the overall frequency of sectoring was reduced. Instability of the 403 site involved multiple nonallelic recombination events that led to the formation of a monocentric translocation. This translocation remained unstable, frequently undergoing either loss or recombination events linked to the translocation junction. These results suggest a model in which instability initiates at specific chromosomal sites that stall replication forks. Forks not stabilized by checkpoint proteins break and undergo multiple rounds of nonallelic recombination to form translocations. Some translocations remain unstable because they join two "incompatible" chromosomal regions. Cycles of instability of this normal yeast chromosome may be relevant to chromosome instability of mammalian fragile sites and of chromosomes in cancer cells. © 2006 by Cold Spring Harbor Laboratory Press.
- Weinert, T., & Weinert, T. -. (2005). Do telomeres ask checkpoint proteins: "gimme shelter-in"?. Developmental cell, 9(6).More infoTelomeres are complicated structures designed to allow one thing and avoid another. They allow replication of chromosome ends, an issue mostly about telomerase, which we seem to understand (though details of its regulation are works in progress). Telomeres must also avoid being detected as DNA breaks. This is important for two reasons: DNA breaks activate checkpoints that cause arrest of cell division, and DNA breaks engage repair machinery. Clearly, normal telomeres neither activate cell cycle arrest nor allow themselves to be repaired; arrest blocks cell division, and repair fuses chromosomes.
- Weinert, T., Michelson, R. J., Rosenstein, S., & Weinert, T. -. (2005). A telomeric repeat sequence adjacent to a DNA double-stranded break produces an anticheckpoint. Genes & development, 19(21).More infoTelomeres are complex structures that serve to protect chromosome ends. Here we provide evidence that in Saccharomyces cerevisiae telomeres may contain an anticheckpoint activity that prevents chromosome ends from signaling cell cycle arrest. We found that an internal tract of telomeric repeats inhibited DNA damage checkpoint signaling from adjacent double-strand breaks (DSBs); cell cycle arrest lasted 8-12 h from a normal DSB, whereas it lasted only 1-2 h from a DSB adjacent to a telomeric repeat. The shortened or abridged arrest was not the result of DNA repair, nor reduced amounts of single-stranded DNA, nor of adaptation. The molecular identity of this telomere repeat-associated anticheckpoint activity is unknown, though it is not dependent upon telomerase or telomere-proximal gene silencing. The anticheckpoint may inhibit the ATR yeast ortholog Mec1 because Rad9 and Rad53 became dephosphorylated and inactivated during the abridged arrest. The anticheckpoint acts regionally; it inhibited signaling from DNA breaks up to 0.6 kb away from the telomeric repeat but not from a DSB present on a separate chromosome. We propose that after formation of the DSB near the telomeric repeat, a mature telomere forms in 1-2 h, and the telomere then contains proteins that inhibit checkpoint signaling from nearby DNA breaks.
- Jia, X., Weinert, T., & Lydall, D. (2004). Mec1 and Rad53 Inhibit Formation of Single-Stranded DNA at Telomeres of Saccharomyces cerevisiae cdc13-1 Mutants. Genetics, 166(2), 753-764.More infoPMID: 15020465;PMCID: PMC1470748;Abstract: Here we examine the roles of budding-yeast checkpoint proteins in regulating degradation of dsDNA to ssDNA at unprotected telomeres (in Cdc13 telomere-binding protein defective strains). We find that Rad17, Mec3, as well as Rad24, members of the putative checkpoint clamp loader (Rad24) and sliding clamp (Rad17, Mec3) complexes, are important for promoting degradation of dsDNA in and near telomere repeats. We find that Mec1, Rad53, as well as Rad9, have the opposite role: they inhibit degradation. Downstream checkpoint kinases Chk1 and Dun1 play no detectable role in either promoting degradation or inhibiting it. These data suggest, first, that the checkpoint sliding clamp regulates and/or recruits some nucleases for degradation, and, second, that Mec1 activates Rad9 to activate Rad53 to inhibit degradation. Further analysis shows that Rad9 inhibits ssDNA generation by both Mec1/Rad53-dependent and -independent pathways. Exo1 appears to be targeted by the Mec1/Rad53-dependent pathway. Finally, analysis of double mutants suggests a minor role for Mec1 in promoting Rad24-dependent degradation of dsDNA. Thus, checkpoint proteins orchestrate carefully ssDNA production at unprotected telomeres.
- Weinert, T., Nyberg, K. A., Michelson, R. J., Putnam, C. W., & Weinert, T. -. (2002). Toward maintaining the genome: DNA damage and replication checkpoints. Annual review of genetics, 36.More infoDNA checkpoints play a significant role in cancer pathology, perhaps most notably in maintaining genome stability. This review summarizes the genetic and molecular mechanisms of checkpoint activation in response to DNA damage. The major checkpoint proteins common to all eukaryotes are identified and discussed, together with how the checkpoint proteins interact to induce arrest within each cell cycle phase. Also discussed are the molecular signals that activate checkpoint responses, including single-strand DNA, double-strand breaks, and aberrant replication forks. We address the connection between checkpoint proteins and damage repair mechanisms, how cells recover from an arrest response, and additional roles that checkpoint proteins play in DNA metabolism. Finally, the connection between checkpoint gene mutation and genomic instability is considered.
- Weinert, T., Little, E., Shanks, L., Admire, A., Gardner, R., Putnam, C., Michelson, R., Nyberg, K., & Sundareshan, P. (2000). Details and concerns regarding the G2/M DNA damage checkpoint in budding yeast. Cold Spring Harbor Symposia on Quantitative Biology, 65, 433-441.More infoPMID: 12760059;
- Weinert, T., Michelson, R. J., & Weinert, T. -. (2000). Closing the gaps among a web of DNA repair disorders. BioEssays : news and reviews in molecular, cellular and developmental biology, 22(11).More infoAs recently as six years ago, three human diseases with similar phenotypes were mistakenly believed to be caused by a single genetic defect. The three diseases, Ataxia-telangiectasia, Nijmegen breakage syndrome, and an AT-like disorder are now known, however, to have defects in three separate genes: ATM, NBS1, and MRE11. Furthermore, new recent studies have shown now that all three gene products interact; the ATM kinase phosphorylates NBS1, which, in turn, associates with MRE11 to regulate DNA repair. Remarkably or expectedly, depending on one's point of view, the similarity in disease phenotypes is evidently due to defects in a common DNA repair pathway.
- Grushcow, J. M., Holzen, T. M., Park, K. J., Weinert, T., Lichten, M., & Bishop, D. K. (1999). Saccharomyces cerevisiae checkpoint genes MEC1, RAD17 and RAD24 are required for normal meiotic recombination partner choice. Genetics, 153(2), 607-620.More infoPMID: 10511543;PMCID: PMC1460798;Abstract: Checkpoint gene function prevents meiotic progression when recombination is blocked by mutations in the recA homologue DMC1. Bypass of dmc1 arrest by mutation of the DNA damage checkpoint genes MEC1, RAD17, or RAD24 results in a dramatic loss of spore viability, suggesting that these genes play an important role in monitoring the progression of recombination. We show here that the role of mitotic checkpoint genes in meiosis is not limited to maintaining arrest in abnormal meioses; mec1-1, rad24, and rad17 single mutants have additional meiotic defects. All three mutants display Zip1 polycomplexes in two- to threefold more nuclei than observed in wild- type controls, suggesting that synapsis may be aberrant. Additionally, all three mutants exhibit elevated levels of ectopic recombination in a novel physical assay. rad17 mutants also alter the fraction of recombination events that are accompanied by an exchange of flanking markers. Crossovers are associated with up to 90% of recombination events for one pair of alleles in rad17, as compared with 65% in wild type. Meiotic progression is not required to allow ectopic recombination in rad17 mutants, as it still occurs at elevated levels in ndt80 mutants that arrest in prophase regardless of checkpoint signaling. These observations support the suggestion that MEC1, RAD17, and RAD24, in addition to their proposed monitoring function, act to promote normal meiotic recombination.
- Michelson, R., & Weinert, T. (1999). Sensor-less checkpoint activation?. Nature Cell Biology, 1(7), 177-179.More infoPMID: 10559997;Abstract: DNA damage activates a set of proteins whose job is to delay the cell cycle until the damage is repaired. This process was thought to involve the detection of damage by sensor proteins, which transmit a signal to a key protein kinase and thence to downstream targets. Unexpectedly, damage can also activate the kinase directly.
- Weinert, T., & Lundblad, V. (1999). Forever hopeful relations: Chromatin, telomeres and checkpoints. Nature Genetics, 21(2), 151-152.More infoPMID: 9988261;
- Weinert, T., Gardner, R., Putnam, C. W., & Weinert, T. -. (1999). RAD53, DUN1 and PDS1 define two parallel G2/M checkpoint pathways in budding yeast. The EMBO journal, 18(11).More infoEukaryotic checkpoint genes regulate multiple cellular responses to DNA damage. In this report, we examine the roles of budding yeast genes involved in G2/M arrest and tolerance to UV exposure. A current model posits three gene classes: those encoding proteins acting on damaged DNA (e.g. RAD9 and RAD24), those transducing a signal (MEC1, RAD53 and DUN1) or those participating more directly in arrest (PDS1). Here, we define important features of the pathways subserved by those genes. MEC1, which we find is required for both establishment and maintenance of G2/M arrest, mediates this arrest through two parallel pathways. One pathway requires RAD53 and DUN1 (the 'RAD53 pathway'); the other pathway requires PDS1. Each pathway independently contributes approximately 50% to G2/M arrest, effects demonstrable after cdc13-induced damage or a double-stranded break inflicted by the HO endonuclease. Similarly, both pathways contribute independently to tolerance of UV irradiation. How the parallel pathways might interact ultimately to achieve arrest is not yet understood, but we do provide evidence that neither the RAD53 nor the PDS1 pathway appears to maintain arrest by inhibiting adaptation. Instead, we think it likely that both pathways contribute to establishing and maintaining arrest.
- Weinert, T., & Weinert, T. -. (1998). DNA damage and checkpoint pathways: molecular anatomy and interactions with repair. Cell, 94(5).
- Weinert, T., & Weinert, T. -. (1998). DNA damage checkpoints update: getting molecular. Current opinion in genetics & development, 8(2).More infoEukaryotic checkpoint controls impose delays in the cell cycle in response to DNA damage or defects in DNA replication. Genetic and physiological studies in budding yeast have identified key genes and defined genetic pathways involved in checkpoint-mediated responses. Recent studies now lead to biochemical models that explain at least in part the arrest in G1 and delays during DNA replication after damage. Though progress in checkpoint controls has indeed been rapid, several observations identify puzzling aspects of checkpoint controls with few plausible explanations.
- Weinert, T., & Weinert, T. -. (1997). A DNA damage checkpoint meets the cell cycle engine. Science (New York, N.Y.), 277(5331).
- Weinert, T., & Weinert, T. -. (1997). Yeast checkpoint controls and relevance to cancer. Cancer surveys, 29.More infoCheckpoint controls arrest cells with defects in DNA replication or DNA damage. For several reasons, checkpoint controls may be relevant to ontogeny and treatment of cancer. Firstly, mutations in two human genes, TP53 and ATM, give rise to cellular defects in cell cycle checkpoints and are associated with cancer. Secondly, although checkpoint defects potentially render the cell damage sensitive, they may do so only in combination with other defects in the cell's response to damage. Therefore, manipulation of checkpoint defects, requiring a description of normal and mutant pathways, will be required for this type of therapeutic approach. Those pathways are being described in yeast cells. In budding yeast, the study of checkpoint genes has led to the view that these genes have many roles in the cellular responses to DNA damage, including roles in arrest in multiple stages of cell cycle, in transcriptional induction of repair genes, in DNA repair itself and additionally some undefined role in DNA replication. The checkpoint pathways and proteins that carry out these responses may consist of sensor proteins that detect damage, signaller proteins that transduce an inhibitory signal and target proteins that are altered to arrest cell division (or cause other changes in cell behaviour). Yeast genes that may act at each step have been identified, leading to a working model of checkpoint pathways. An initial step in the pathway may involve the processing of damage to an intermediate that signals arrest and acts in DNA repair. Human checkpoint pathways may have defects in processing damage as well.
- Weinert, T., Kim, S., & Weinert, T. -. (1997). Characterization of the checkpoint gene RAD53/MEC2 in Saccharomyces cerevisiae. Yeast (Chichester, England), 13(8).More infoSaccharomyces cerevisiae cells carrying mutations in RAD53/MEC2 fail to arrest in the S phase when DNA replication is blocked (the S/M checkpoint) or in the G2 phase when DNA is damaged (the G2/M checkpoint). We isolated and determined the DNA sequence of RAD53 and found that it is identical to the SPK1 gene previously identified by Stern et al. (1991). In addition to its checkpoint functions, we show here that RAD53 is essential for cell viability because null mutants are inviable. Weak genomic suppressors of the essential function do arise frequently, though they do not suppress the checkpoint defects of the null mutant. This genetically separates the essential and checkpoint functions. We show genetically that the protein kinase domain is essential for all RAD53-dependent functions tested because a site-specific mutation that inactivates the protein kinase activity results in a mutant phenotype indistinguishable from that of a null mutant. Overexpression of RAD53, or its kinase domain alone, resulted in a delay in cell-cycle progression that required the intact kinase function. The cell-cycle delay did not require any of the checkpoint genes tested (e.g. rad9 or mecl), indicating that the cell-cycle delay is either unrelated to the checkpoint responses, or that it occurs constitutively because RAD53 acts further downstream of the checkpoint genes tested. Finally, elimination of sequences in the promoter region of RAD53 revealed complex regulatory elements.
- Weinert, T., Lydall, D., & Weinert, T. -. (1997). G2/M checkpoint genes of Saccharomyces cerevisiae: further evidence for roles in DNA replication and/or repair. Molecular & general genetics : MGG, 256(6).More infoWe have cloned, sequenced and disrupted the checkpoint genes RAD17, RAD24 and MEC3 of Saccharomyces cerevisiae. Mec3p shows no strong similarity to other proteins currently in the database. Rad17p is similar to Rec1 from Ustilago maydis, a 3' to 5' DNA exonuclease/checkpoint protein, and the checkpoint protein Rad1p from Schizosaccharomyces pombe (as we previously reported). Rad24p shows sequence similarity to replication factor C (RFC) subunits, and the S. pombe Rad17p checkpoint protein, suggesting it has a role in DNA replication and/or repair. This hypothesis is supported by our genetic experiments which show that overexpression of RAD24 strongly reduces the growth rate of yeast strains that are defective in the DNA replication/repair proteins Rfc1p (cdc44), DNA pol alpha (cdc17) and DNA pol delta (cdc2) but has much weaker effects on cdc6, cdc9, cdc15 and CDC4 strains. The idea that RAD24 overexpression induces DNA damage, perhaps by interfering with replication/repair complexes, is further supported by our observation that RAD24 overexpression increases mitotic chromosome recombination in CDC4 strains. Although RAD17, RAD24 and MEC3 are not required for cell cycle arrest when S phase is inhibited by hydroxyurea (HU), they do contribute to the viability of yeast cells grown in the presence of HU, possibly because they are required for the repair of HU-induced DNA damage. In addition, all three are required for the rapid death of cdc13 rad9 mutants. All our data are consistent with models in which RAD17, RAD24 and MEC3 are coordinately required for the activity of one or more DNA repair pathways that link DNA damage to cell cycle arrest.
- Weinert, T., Lydall, D., & Weinert, T. -. (1997). Use of cdc13-1-induced DNA damage to study effects of checkpoint genes on DNA damage processing. Methods in enzymology, 283.
- Lydall, D., & Weinert, T. (1996). From DNA damage to cell cycle arrest and suicide: A budding yeast perspective. Current Opinion in Genetics and Development, 6(1), 4-11.More infoPMID: 8791492;Abstract: Eukaryotic checkpoint control genes are important for cell cycle delay, DNA repair and cell suicide after DNA is damaged. Recent studies in budding yeast show how the participation of checkpoint control proteins in DNA metabolism could lead to all three of these outcomes.
- Lydall, D., Nikolsky, Y., Bishop, D. K., & Weinert, T. (1996). A meiotic recombination checkpoint controlled by mitotic checkpoint genes. Nature, 383(6603), 841-844.More infoAbstract: IN budding yeast, meiotic recombination occurs at about 200 sites per cell and involves DNA double-strand break (DSB) intermediates. Here we provide evidence that a checkpoint control requiring the mitotic DNA-damage checkpoint genes RAD17, RAD24 and MEC1 ensures that meiotic recombination is complete before the first ratiotic division (MI). First, RAD17, RAD24 and MEC1 are required for the meiotic arrest caused by blocking the repair of DSBs with a mutation in the recA homologue DMC1. Second, mec1 and rad24 single routants (DMC1+) appear to undergo MI before all recombination events are complete. Curiously, the mitosis-specific checkpoint gene RAD9 is not required for meiotic arrest of dmc1 mutants. This shows that although mitotic and meiotic control mechanisms are related, they differ significantly. Rad17 and Rad24 proteins may contribute directly to formation of an arrest signal by association with single-strand DNA in mitosis and meiosis.
- Weinert, T., Kiser, G. L., & Weinert, T. -. (1996). Distinct roles of yeast MEC and RAD checkpoint genes in transcriptional induction after DNA damage and implications for function. Molecular biology of the cell, 7(5).More infoIn eukaryotic cells, checkpoint genes cause arrest of cell division when DNA is damaged or when DNA replication is blocked. In this study of budding yeast checkpoint genes, we identify and characterize another role for these checkpoint genes after DNA damage-transcriptional induction of genes. We found that three checkpoint genes (of six genes tested) have strong and distinct roles in transcriptional induction in four distinct pathways of regulation (each defined by induction of specific genes). MEC1 mediates the response in three transcriptional pathways, RAD53 mediates two of these pathways, and RAD17 mediates but a single pathway. The three other checkpoint genes (including RAD9) have small (twofold) but significant roles in transcriptional induction in all pathways. One of the pathways that we identify here leads to induction of MEC1 and RAD53 checkpoint genes themselves. This suggests a positive feedback circuit that may increase the cell's ability to respond to DNA damage. We make two primary conclusions from these studies. First, MEC1 appears to be the key regulator because it is required for all responses (both transcriptional and cell cycle arrest), while other genes serve only a subset of these responses. Second, the two types of responses, transcriptional induction and cell cycle arrest, appear distinct because both require MEC1 yet only cell cycle arrest requires RAD9. These and other results were used to formulate a working model of checkpoint gene function that accounts for roles of different checkpoint genes in different responses and after different types of damage. The conclusion that the yeast MEC1 gene is a key regulator also has implications for the role of a putative human homologue, the ATM gene.
- Weinert, T., Lydall, D., & Weinert, T. -. (1996). From DNA damage to cell cycle arrest and suicide: a budding yeast perspective. Current opinion in genetics & development, 6(1).More infoEukaryotic checkpoint control genes are important for cell cycle delay, DNA repair and cell suicide after DNA is damaged. Recent studies in budding yeast show how the participation of checkpoint control proteins in DNA metabolism could lead to all three of these outcomes.
- Lydall, D., & Weinert, T. (1995). Yeast checkpoint genes in DNA damage processing: Implications for repair and arrest. Science, 270(5241), 1486-1491.More infoPMID: 7491494;Abstract: Yeast checkpoint control genes were found to affect processing of DNA damage as well as cell cycle arrest. An assay that measures DNA damage processing in vivo showed that the checkpoint genes RAD17, RAD24, and MEC3 activated an exonuclease that degrades DNA. The degradation is probably a direct consequence of checkpoint protein function, because RAD17 encodes a putative 3′-5′ DNA exonuclease. Another checkpoint gene, RAD9, had a different role: It inhibited the degradation by RAD17, RAD24, and MEC3. A model of how processing of DNA damage may be linked to both DNA repair and cell cycle arrest is proposed.
- Weinert, T., Kiser, G. L., & Weinert, T. -. (1995). GUF1, a gene encoding a novel evolutionarily conserved GTPase in budding yeast. Yeast (Chichester, England), 11(13).More infoWhile sequencing a region of chromosome IV adjacent to the checkpoint gene MEC3, we identified a gene we call GUF1 (GTPase of Unknown Function), which predicts a 586 amino acid GTPase of the elongation factor-type class. The predicted Guf1p protein bears striking sequence similarity to both LepA from Escherichia coli (43% identical) and LK1236.1 from Caenorhabditis elegans (42% identical). Analysis of both a guf1 delta deletion and a putative constitutive-activating mutant (GUF1HG) revealed that GUF1 is not essential nor did mutant cells reveal any marked phenotype.
- Weinert, T., Lydall, D., & Weinert, T. -. (1995). Yeast checkpoint genes in DNA damage processing: implications for repair and arrest. Science (New York, N.Y.), 270(5241).More infoYeast checkpoint control genes were found to affect processing of DNA damage as well as cell cycle arrest. An assay that measures DNA damage processing in vivo showed that the checkpoint genes RAD17, RAD24, and MEC3 activated an exonuclease that degrades DNA. The degradation is probably a direct consequence of checkpoint protein function, because RAD17 encodes a putative 3'-5' DNA exonuclease. Another checkpoint gene, RAD9, had a different role: It inhibited the degradation by RAD17, RAD24, and MEC3. A model of how processing of DNA damage may be linked to both DNA repair and cell cycle arrest is proposed.
- Hartwell, L., Weinert, T., Kadyk, L., & Garvik, B. (1994). Cell cycle checkpoints, genomic integrity, and cancer. Cold Spring Harbor Symposia on Quantitative Biology, 59, 259-263.More infoPMID: 7587077;
- Weinert, T. A., Kiser, G. L., & Hartwell, L. H. (1994). Mitotic checkpoint genes in budding yeast and the dependence of mitosis on DNA replication and repair. Genes and Development, 8(6), 652-665.More infoPMID: 7926756;Abstract: In eukaryotes a cell-cycle control termed a checkpoint causes arrest in the S or G2 phases when chromosomes are incompletely replicated or damaged. Previously, we showed in budding yeast that RAD9 and RAD17 are checkpoint genes required for arrest in the G2 phase after DNA damage. Here, we describe a genetic strategy that identified four additional checkpoint genes that act in two pathways. Both classes of genes are required for arrest in the G2 phase after DNA damage, and one class of genes is also required for arrest in S phase when DNA replication is incomplete. The G2-specific genes include MEC3 (for mitosis entry checkpoint), RAD9, RAD17, and RAD24. The genes common to both S phase and G2 phase pathways are MEC1 and MEC2. The MEC2 gene proves to be identical to the RAD53 gene. Checkpoint mutants were identified by their interactions with a temperature-sensitive allele of the cell division cycle gene CDC13; cdc13 mutants arrested in G2 and survived at the restrictive temperature, whereas all cdc13 checkpoint double mutants failed to arrest in G2 and died rapidly at the restrictive temperature. The cell-cycle roles of the RAD and MEC genes were examined by combination of rad and mec mutant alleles with 10 cdc mutant alleles that arrest in different stages of the cell cycle at the restrictive temperature and by the response of rad and mec mutant alleles to DNA damaging agents and to hydroxyurea, a drug that inhibits DNA replication. We conclude that the checkpoint in budding yeast consists of overlapping S-phase and G2-phase pathways that respond to incomplete DNA replication and/or DNA damage and cause arrest of cells before mitosis.
- Weinert, T. A., & Hartwell, L. H. (1993). Cell cycle arrest of cdc mutants and specificity of the RAD9 checkpoint. Genetics, 134(1), 63-80.More infoPMID: 8514150;PMCID: PMC1205445;Abstract: In eucaryotes a cell cycle control called a checkpoint ensures that mitosis occurs only after chromosomes are completely replicated and any damage is repaired. The function of this checkpoint in budding yeast requires the RAD9 gene. Here we examine the role of the RAD9 gene in the arrest of the 12 cell division cycle (cdc) mutants, temperature-sensitive lethal mutants that arrest in specific phases of the cell cycle at a restrictive temperature. We found that in four cdc mutants the cdc rad9 cells failed to arrest after a shift to the restrictive temperature, rather they continued cell division and died rapidly, whereas the cdc RAD cells arrested and remained viable. The cell cycle and genetic phenotypes of the 12 cdc RAD mutants indicate the function of the RAD9 checkpoint is phase-specific and signal-specific. First, the four cdc RAD mutants that required RAD9 each arrested in the late S/G2 phase after a shift to the restrictive temperature when DNA replication was complete or nearly complete, and second, each leaves DNA lesions when the CDC gene product is limiting for cell division. Three of the four CDC genes are known to encode DNA replication enzymes. We found that the RAD17 gene is also essential for the function of the RAD9 checkpoint because it is required for phase-specific arrest of the same four cdc mutants. We also show that both X- or UV-irradiated cells require the RAD9 and RAD17 genes for delay in the G2 phase. Together, these results indicate that the RAD9 checkpoint is apparently activated only by DNA lesions and arrests cell division only in the late S/G2 phase.
- Weinert, T., & Lydall, D. (1993). Cell cycle checkpoints, genetic instability and cancer. Seminars in Cancer Biology, 4(2), 129-140.More infoPMID: 8513148;Abstract: During the cell cycle, the order of events is maintained by controls termed checkpoints. Two checkpoints are sensitive to DNA damage, one that acts before mitosis and a second that acts before DNA replication. This is relevant to cancer because checkpoint mutants show genetic instability, and such instability is characteristic of many cancers. Studies of checkpoints in normal and cancer cells suggest a mechanistic relationship to the central cell cycle control p34CDC2 and its regulators. We suggest how mutations in these genes and those with a role in DNA metabolism may affect the function of checkpoints. A further link between checkpoints and cancer may be the p53 protein, which appears to Junction at the G1-S checkpoint. Consideration of checkpoints may provide more effective means for cancer treatment. © 1993 Academic Press Ltd.
- Weinert, T., & Weinert, T. -. (1992). Dual cell cycle checkpoints sensitive to chromosome replication and DNA damage in the budding yeast Saccharomyces cerevisiae. Radiation research, 132(2).More infoIn eucaryotic cells chromosomes must be fully replicated and repaired before mitosis begins. Genetic studies indicate that this dependence of mitosis on completion of DNA replication and DNA repair derives from a negative control called a checkpoint which somehow checks for replication and DNA damage and blocks cell entry into mitosis. Here we summarize our current understanding of the genetic components of the cell cycle checkpoint in budding yeast. Mutants were identified and their phase and signal specificity tested primarily through interactions of the arrest-defective mutants with cell division cycle mutants. The results indicate that dual checkpoint controls exist in budding yeast, one control sensitive to inhibition of DNA replication (S-phase checkpoint), and a distinct but overlapping control sensitive to DNA repair (G2 checkpoint). Six genes are required for arrest in G2 phase after DNA damage (RAD9, RAD17, RAD24, MEC1, MEC2, and MEC3), and two of these are also essential for arrest in S phase when DNA replication is blocked (MEC1 and MEC2).
- Brown, M., Garvik, B., Hartwell, L., Kadyk, L., Seeley, T., & Weinert, T. (1991). Fidelity of mitotic chromosome transmission. Cold Spring Harbor Symposia on Quantitative Biology, 56, 359-365.More infoPMID: 1819497;
- Weinert, T. A., & Hartwell, L. H. (1990). Characterization of RAD9 of Saccharomyces cerevisiae and evidence that its function acts posttranslationally in cell cycle arrest after DNA damage. Molecular and Cellular Biology, 10(12), 6554-6564.More infoPMID: 2247073;PMCID: PMC362932;Abstract: In eucaryotic cells, incompletely replicated or damaged chromosomes induce cell cycle arrest in G2 before mitosis, and in the yeast Saccharomyces cerevisiae the RAD9 gene is essential for the cell cycle arrest (T. A. Weinert and L. H. Hartwell, Science 241:317-322, 1988). In this report, we extend the analysis of RAD9-dependent cell cycle control. We found that both induction of RAD9-dependent arrest in G2 and recovery from arrest could occur in the presence of the protein synthesis inhibitor cycloheximide, showing that the mechanism of RAD9-dependent control involves a posttranslational mechanism(s). We have isolated and determined the DNA sequence of the RAD9 gene, confirming the DNA sequence reported previously (R. H. Schiestl, P. Reynolds, S. Prakash, and L. Prakash, Mol. Cell. Biol. 9:1882-1886, 1989). The predicted protein sequence for the Rad9 protein bears no similarity to sequences of known proteins. We also found that synthesis of the RAD9 transcript in the cell cycle was constitutive and not induced by X-irradiation. We constructed yeast cells containing a complete deletion of the RAD9 gene; the rad9 null mutants were viable, sensitive to X- and UV irradiation, and defective for cell cycle arrest after DNA damage. Although Rad+ and rad9Δ cells had similar growth rates and cell cycle kinetics in unirradiated cells, the spontaneous rate of chromosome loss (in unirradiated cells) was elevated 7- to 21-fold in rad9Δ cells. These studies show that in the presence of induced or endogenous DNA damage, RAD9 is a negative regulator that inhibits progression from G2 in order to preserve cell viability and to maintain the fidelity of chromosome transmission.
- Hartwell, L. H., & Weinert, T. A. (1989). Checkpoints: controls that ensure the order of cell cycle events. Science, 246(4930), 629-634.More infoPMID: 2683079;Abstract: The events of the cell cycle of most organisms are ordered into dependent pathways in which the initiation of late events is dependent on the completion of early events. In eukaryotes, for example, mitosis is dependent on the completion of DNA synthesis. Some dependencies can be relieved by mutation (mitosis may then occur before completion of DNA synthesis), suggesting that the dependency is due to a control mechanism and not an intrinsic feature of the events themselves. Control mechanisms enforcing dependency in the cell cycle are here called checkpoints. Elimination of the checkpoints may result in cell death, infidelity in the distribution of chromosomes or other organelles, or increased susceptibility to environmental perturbations such as DNA damaging agents. It appears that some checkpoints are eliminated during the early embryonic development of some organisms; this fact may pose special problems for the fidelity of embryonic cell division.
- Weinert, T., & Hartwell, L. (1989). Control of G2 delay by the RAD9 gene of Saccharomyces cerevisiae. Journal of Cell Science, 94(SUPPL. 12), 145-148.More infoPMID: 2699734;Abstract: In response to DNA damage, Saccharomyces cerevisiae cells arrest the cell cycle in the G2 phase. Arrest is defective in rad9 mutants; rad9 cells divide and die without repairing the damage. Several cell cycle mutants that are defective in DNA replication arrest in G2 at the restrictive temperature; this arrest is due to the RAD9 control function. Thus RAD9 is responsible for the fact that mitosis is normally dependent upon DNA replication, a function we term a 'checkpoint'. Four additional genes have been identified that are also components of the RAD9 checkpoint.
- Weinert, T. A., & Hartwell, L. H. (1988). The RAD9 gene controls the cell cycle response to DNA damage in Saccharomyces cerevisiae. Science, 241(4863), 317-322.More infoPMID: 3291120;Abstract: Cell division is arrested in many organisms in response to DNA damage. Examinations of the genetic basis for this response in the yeast Saccharomyces cerevisiae indicate that the RAD9 gene product is essential for arrest of cell division induced by DNA damage. Wild-type haploid cells irradiated with x-rays either arrest or delay cell division in the G2 phase of cell cycle. Irradiated G1 and M phase haploid cells arrest irreversibly in G2 and die, whereas irradiated G2 phase haploid cells delay in G2 for a time proportional to the extent of damage before resuming cell division. In contrast, irradiated rad9 cells in any phase of the cycle do not delay cell division in G2, but continue to divide for several generations and die. However, efficient DNA repair can occur in irradiated rad9 cells if irradiated cells are blocked for several hours in G2 by treatment with a microtubule poison. The RAD9-dependent response detects potentially lethal DNA damage and causes arrest of cells in G2 until such damage is repaired.
Presentations
- Weinert, T. (2018, July). Yeast Genome Instability. FASEB Yeast Chromosome and Cell Cycle. Colorado: FASEB.
- Weinert, T. (2015, Feb). Genome Instability in Yeast. Invited Speaker North Dakota University.
- Weinert, T. (2015, February). Genome Instability in Yeast. Duke Graduate Student Invited Speaker.
- Weinert, T. (2014, April). Genome Instability in Yeast. University of Arizona Joint Seminar Program.
- Weinert, T. (2014, January 2014). Genome Instability in Yeast. Invited Speaker. Santa Cruz.
- Weinert, T. (2013, April). Genome Instability in Yeast. Salk Institute departmental talk.