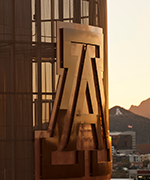
Jacob C Schwartz
- Associate Research Professor, Pharmacology - (Research Series Track)
- Assistant Professor, Chemistry and Biochemistry - Med
- Member of the Graduate Faculty
- Associate Professor, Cancer Biology - GIDP
- Associate Professor, Neuroscience - GIDP
- Associate Professor, BIO5 Institute
Contact
- (940) 367-8186
- Life Sciences North, Rm. LSN649
- Tucson, AZ 85724
- jcschwartz@arizona.edu
Awards
- Kavli Scholarship
- Kavli Foundation, National Academy of Sciences, Fall 2015
Interests
No activities entered.
Courses
2025-26 Courses
-
Intro to Pharmacology
PHCL 412 (Fall 2025) -
Intro to Pharmacology
PHCL 512 (Fall 2025) -
Sci Writ, Present & Bioethics
PHCL 595B (Fall 2025)
2024-25 Courses
-
Directed Research
PHCL 692 (Spring 2025) -
Dissertation
PHCL 920 (Spring 2025) -
Dissertation
PHSC 920 (Spring 2025) -
Honors Thesis
BIOC 498H (Spring 2025) -
Research Conference
PCOL 695A (Spring 2025) -
Directed Research
PHCL 692 (Fall 2024) -
Dissertation
PHCL 920 (Fall 2024) -
Dissertation
PHSC 920 (Fall 2024) -
Honors Thesis
BIOC 498H (Fall 2024) -
Intro to Pharmacology
PHCL 412 (Fall 2024) -
Intro to Pharmacology
PHCL 512 (Fall 2024) -
Research Conference
PCOL 695A (Fall 2024) -
Sci Writ, Present & Bioethics
PHCL 595B (Fall 2024)
2023-24 Courses
-
Directed Research
PHCL 392 (Spring 2024) -
Directed Research
PHCL 692 (Spring 2024) -
Dissertation
BIOC 920 (Spring 2024) -
Dissertation
PHCL 920 (Spring 2024) -
Exchange Chemical Info
CHEM 695B (Spring 2024) -
Research
PHSC 900 (Spring 2024) -
Directed Research
PHCL 692 (Fall 2023) -
Dissertation
BIOC 920 (Fall 2023) -
Dissertation
PHCL 920 (Fall 2023) -
Exchange Chemical Info
CHEM 695B (Fall 2023) -
Intro to Pharmacology
PHCL 412 (Fall 2023) -
Intro to Pharmacology
PHCL 512 (Fall 2023) -
Research
PHSC 900 (Fall 2023) -
Research Conference
PCOL 695A (Fall 2023) -
Sci Writ, Present & Bioethics
PHCL 595B (Fall 2023)
2022-23 Courses
-
Directed Research
PHCL 692 (Spring 2023) -
Directed Research
PHSC 792A (Spring 2023) -
Dissertation
BIOC 920 (Spring 2023) -
Dissertation
PHCL 920 (Spring 2023) -
Exchange Chemical Info
CHEM 695B (Spring 2023) -
Research
PHSC 900 (Spring 2023) -
Directed Research
PHCL 692 (Fall 2022) -
Dissertation
BIOC 920 (Fall 2022) -
Dissertation
PHCL 920 (Fall 2022) -
Research Conference
PCOL 695A (Fall 2022)
2021-22 Courses
-
Designing Drugs
PHCL 460 (Spring 2022) -
Directed Research
BIOC 492 (Spring 2022) -
Dissertation
BIOC 920 (Spring 2022) -
Exchange Chemical Info
CHEM 695B (Spring 2022) -
Honors Directed Research
NSCS 492H (Spring 2022) -
Honors Thesis
NSCS 498H (Spring 2022) -
Nucleic Acids, Metab & Signal
BIOC 568 (Spring 2022) -
Nucleic Acids, Metab & Signal
GENE 568 (Spring 2022) -
Seminar in Biochemistry
BIOC 696D (Spring 2022) -
Senior Capstone
BIOC 498 (Spring 2022) -
Dissertation
BIOC 920 (Fall 2021) -
Metabolic Biochemistry
BIOC 385 (Fall 2021) -
Seminar in Biochemistry
BIOC 696D (Fall 2021) -
Senior Capstone
BIOC 498 (Fall 2021)
2020-21 Courses
-
Dissertation
BIOC 920 (Spring 2021) -
Exchange Chemical Info
CHEM 695B (Spring 2021) -
Honors Directed Research
NSCS 392H (Spring 2021) -
Honors Thesis
BIOC 498H (Spring 2021) -
Journal Club
BIOC 595B (Spring 2021) -
Nucleic Acids, Metab & Signal
BIOC 568 (Spring 2021) -
Research
BIOC 900 (Spring 2021) -
Seminar in Biochemistry
BIOC 696D (Spring 2021) -
Senior Capstone
BIOC 498 (Spring 2021) -
Thesis
BIOC 910 (Spring 2021) -
Directed Research
BIOC 392 (Fall 2020) -
Directed Research
MCB 792 (Fall 2020) -
Dissertation
BIOC 920 (Fall 2020) -
Exchange Chemical Info
CHEM 695B (Fall 2020) -
Honors Thesis
BIOC 498H (Fall 2020) -
Research
BIOC 900 (Fall 2020) -
Seminar in Biochemistry
BIOC 696D (Fall 2020) -
Senior Capstone
BIOC 498 (Fall 2020)
2019-20 Courses
-
Biochem of Nucleic Acids
BIOC 466 (Spring 2020) -
Directed Research
BIOC 392 (Spring 2020) -
Directed Research
BIOC 492 (Spring 2020) -
Directed Rsrch
MCB 392 (Spring 2020) -
Dissertation
BIOC 920 (Spring 2020) -
Dissertation
MCB 920 (Spring 2020) -
Exchange Chemical Info
CHEM 695B (Spring 2020) -
Honors Directed Research
NSCS 392H (Spring 2020) -
Honors Independent Study
BIOC 499H (Spring 2020) -
Honors Independent Study
MCB 399H (Spring 2020) -
Lab Presentations & Discussion
MCB 696A (Spring 2020) -
Nucleic Acids, Metab & Signal
BIOC 568 (Spring 2020) -
Preceptorship
BIOC 491 (Spring 2020) -
Research
BIOC 900 (Spring 2020) -
Seminar in Biochemistry
BIOC 696D (Spring 2020) -
Directed Research
BIOC 392 (Fall 2019) -
Dissertation
BIOC 920 (Fall 2019) -
Dissertation
MCB 920 (Fall 2019) -
Exchange Chemical Info
CHEM 695B (Fall 2019) -
Honors Independent Study
BIOC 499H (Fall 2019) -
Lab Presentations & Discussion
MCB 696A (Fall 2019) -
Research
BIOC 900 (Fall 2019) -
Seminar in Biochemistry
BIOC 696D (Fall 2019)
2018-19 Courses
-
Biochem of Nucleic Acids
BIOC 466 (Spring 2019) -
Directed Rsrch
MCB 492 (Spring 2019) -
Dissertation
BIOC 920 (Spring 2019) -
Dissertation
MCB 920 (Spring 2019) -
Exchange Chemical Info
CHEM 695B (Spring 2019) -
Intro to Biochem Rsrch
BIOC 296B (Spring 2019) -
Lab Presentations & Discussion
MCB 696A (Spring 2019) -
Preceptorship
BIOC 491 (Spring 2019) -
Research
BIOC 900 (Spring 2019) -
Seminar in Biochemistry
BIOC 696D (Spring 2019) -
Senior Capstone
BIOC 498 (Spring 2019) -
Dissertation
BIOC 920 (Fall 2018) -
Dissertation
MCB 920 (Fall 2018) -
Exchange Chemical Info
CHEM 695B (Fall 2018) -
Honors Directed Research
BIOC 392H (Fall 2018) -
Lab Presentations & Discussion
MCB 696A (Fall 2018) -
Research
BIOC 900 (Fall 2018) -
Seminar in Biochemistry
BIOC 696D (Fall 2018) -
Senior Capstone
BIOC 498 (Fall 2018) -
Thesis
BIOC 910 (Fall 2018)
2017-18 Courses
-
Directed Research
BIOC 492 (Spring 2018) -
Directed Rsrch
MCB 492 (Spring 2018) -
Dissertation
BIOC 920 (Spring 2018) -
Dissertation
MCB 920 (Spring 2018) -
Exchange Chemical Info
CHEM 695B (Spring 2018) -
Honors Independent Study
BIOC 399H (Spring 2018) -
Honors Thesis
BIOC 498H (Spring 2018) -
Intro to Biochem Rsrch
BIOC 296B (Spring 2018) -
Lab Presentations & Discussion
MCB 696A (Spring 2018) -
Research
BIOC 900 (Spring 2018) -
Seminar in Biochemistry
BIOC 696D (Spring 2018) -
Senior Capstone
BIOC 498 (Spring 2018) -
Thesis
BIOC 910 (Spring 2018) -
Directed Research
BIOC 492 (Fall 2017) -
Dissertation
BIOC 920 (Fall 2017) -
Dissertation
MCB 920 (Fall 2017) -
Exchange Chemical Info
CHEM 695B (Fall 2017) -
Honors Independent Study
BIOC 299H (Fall 2017) -
Honors Thesis
BIOC 498H (Fall 2017) -
Lab Presentations & Discussion
MCB 696A (Fall 2017) -
Proteins and Enzymes
BIOC 565 (Fall 2017) -
Research
BIOC 900 (Fall 2017) -
Seminar in Biochemistry
BIOC 696D (Fall 2017) -
Senior Capstone
BIOC 498 (Fall 2017)
2016-17 Courses
-
Directed Research
BIOC 392 (Spring 2017) -
Directed Rsrch
MCB 492 (Spring 2017) -
Dissertation
CHEM 920 (Spring 2017) -
Dissertation
MCB 920 (Spring 2017) -
Exchange Chemical Info
CHEM 695B (Spring 2017) -
Honors Independent Study
BIOC 399H (Spring 2017) -
Honors Independent Study
BIOC 499H (Spring 2017) -
Honors Thesis
BIOC 498H (Spring 2017) -
Intro to Biochem Rsrch
BIOC 296B (Spring 2017) -
Lab Presentations & Discussion
MCB 696A (Spring 2017) -
Research
BIOC 900 (Spring 2017) -
Research
CHEM 900 (Spring 2017) -
Seminar in Biochemistry
BIOC 696D (Spring 2017) -
Senior Capstone
BIOC 498 (Spring 2017) -
Senior Capstone
MCB 498 (Spring 2017) -
Dissertation
MCB 920 (Fall 2016) -
Honors Independent Study
BIOC 499H (Fall 2016) -
Honors Thesis
BIOC 498H (Fall 2016) -
Introduction to Research
MCB 795A (Fall 2016) -
Lab Presentations & Discussion
MCB 696A (Fall 2016) -
Proteins and Enzymes
BIOC 565 (Fall 2016) -
Research
BIOC 900 (Fall 2016) -
Seminar in Biochemistry
BIOC 696D (Fall 2016) -
Senior Capstone
BIOC 498 (Fall 2016) -
Senior Capstone
MCB 498 (Fall 2016)
2015-16 Courses
-
Dissertation
MCB 920 (Spring 2016) -
Intro to Biochem Rsrch
BIOC 296B (Spring 2016) -
Lab Presentations & Discussion
MCB 696A (Spring 2016) -
Research
BIOC 900 (Spring 2016) -
Research
MCB 900 (Spring 2016) -
Seminar in Biochemistry
BIOC 696D (Spring 2016)
Scholarly Contributions
Journals/Publications
- Kuzmina, A., Sadhu, L., Hasanuzzaman, M., Fujinaga, K., Schwartz, J. C., Fackler, O. T., & Taube, R. (2024). Direct and indirect effects of CYTOR lncRNA regulate HIV gene expression. PLoS pathogens, 20(4), e1012172.More infoThe implementation of antiretroviral therapy (ART) has effectively restricted the transmission of Human Immunodeficiency Virus (HIV) and improved overall clinical outcomes. However, a complete cure for HIV remains out of reach, as the virus persists in a stable pool of infected cell reservoir that is resistant to therapy and thus a main barrier towards complete elimination of viral infection. While the mechanisms by which host proteins govern viral gene expression and latency are well-studied, the emerging regulatory functions of non-coding RNAs (ncRNA) in the context of T cell activation, HIV gene expression and viral latency have not yet been thoroughly explored. Here, we report the identification of the Cytoskeleton Regulator (CYTOR) long non-coding RNA (lncRNA) as an activator of HIV gene expression that is upregulated following T cell stimulation. Functional studies show that CYTOR suppresses viral latency by directly binding to the HIV promoter and associating with the cellular positive transcription elongation factor (P-TEFb) to activate viral gene expression. CYTOR also plays a global role in regulating cellular gene expression, including those involved in controlling actin dynamics. Depletion of CYTOR expression reduces cytoplasmic actin polymerization in response to T cell activation. In addition, treating HIV-infected cells with pharmacological inhibitors of actin polymerization reduces HIV gene expression. We conclude that both direct and indirect effects of CYTOR regulate HIV gene expression.
- Lay, M. A., Thompson, V. F., Adelakun, A. D., & Schwartz, J. C. (2024). Ewing Sarcoma Related protein 1 recognizes R-loops by binding DNA forks. Biopolymers, 115(3), e23576.More infoEWSR1 (Ewing Sarcoma Related protein 1) is an RNA binding protein that is ubiquitously expressed across cell lines and involved in multiple parts of RNA processing, such as transcription, splicing, and mRNA transport. EWSR1 has also been implicated in cellular mechanisms to control formation of R-loops, a three-stranded nucleic acid structure consisting of a DNA:RNA hybrid and a displaced single-stranded DNA strand. Unscheduled R-loops result in genomic and transcription stress. Loss of function of EWSR1 functions commonly found in Ewing Sarcoma correlates with high abundance of R-loops. In this study, we investigated the mechanism for EWSR1 to recognize an R-loop structure specifically. Using electrophoretic mobility shift assays (EMSA), we detected the high affinity binding of EWSR1 to substrates representing components found in R-loops. EWSR1 specificity could be isolated to the DNA fork region, which transitions between double- and single-stranded DNA. Our data suggests that the Zinc-finger domain (ZnF) with flanking arginine and glycine rich (RGG) domains provide high affinity binding, while the RNA recognition motif (RRM) with its RGG domains offer improved specificity. This model offers a rational for EWSR1 specificity to encompass a wide range in contexts due to the DNA forks always found with R-loops.
- Lay, M., Lay, M., Thompson, V., Thompson, V., Adelakun, A., Adelakun, A., Schwartz, J., & Schwartz, J. (2024). Ewing Sarcoma Related protein 1 recognizes R-loops by binding DNA forks. Biopolymers, 115(3). doi:10.1002/bip.23576More infoEWSR1 (Ewing Sarcoma Related protein 1) is an RNA binding protein that is ubiquitously expressed across cell lines and involved in multiple parts of RNA processing, such as transcription, splicing, and mRNA transport. EWSR1 has also been implicated in cellular mechanisms to control formation of R-loops, a three-stranded nucleic acid structure consisting of a DNA:RNA hybrid and a displaced single-stranded DNA strand. Unscheduled R-loops result in genomic and transcription stress. Loss of function of EWSR1 functions commonly found in Ewing Sarcoma correlates with high abundance of R-loops. In this study, we investigated the mechanism for EWSR1 to recognize an R-loop structure specifically. Using electrophoretic mobility shift assays (EMSA), we detected the high affinity binding of EWSR1 to substrates representing components found in R-loops. EWSR1 specificity could be isolated to the DNA fork region, which transitions between double- and single-stranded DNA. Our data suggests that the Zinc-finger domain (ZnF) with flanking arginine and glycine rich (RGG) domains provide high affinity binding, while the RNA recognition motif (RRM) with its RGG domains offer improved specificity. This model offers a rational for EWSR1 specificity to encompass a wide range in contexts due to the DNA forks always found with R-loops.
- Thompson, V. F., Wieland, D. R., Mendoza-Leon, V., Janis, H. I., Lay, M. A., Harrell, L. M., & Schwartz, J. C. (2023). Binding of the nuclear ribonucleoprotein family member FUS to RNA prevents R-loop RNA:DNA hybrid structures. The Journal of biological chemistry, 299(10), 105237.More infoThe protein FUS (FUSed in sarcoma) is a metazoan RNA-binding protein that influences RNA production by all three nuclear polymerases. FUS also binds nascent transcripts, RNA processing factors, RNA polymerases, and transcription machinery. Here, we explored the role of FUS binding interactions for activity during transcription. In vitro run-off transcription assays revealed FUS-enhanced RNA produced by a non-eukaryote polymerase. The activity also reduced the formation of R-loops between RNA products and their DNA template. Analysis by domain mutation and deletion indicated RNA-binding was required for activity. We interpret that FUS binds and sequesters nascent transcripts to prevent R-loops from forming with nearby DNA. DRIP-seq analysis showed that a knockdown of FUS increased R-loop enrichment near expressed genes. Prevention of R-loops by FUS binding to nascent transcripts has the potential to affect transcription by any RNA polymerase, highlighting the broad impact FUS can have on RNA metabolism in cells and disease.
- Ahmed, N. S., Harrell, L. M., Wieland, D. R., Lay, M. A., Thompson, V. F., & Schwartz, J. C. (2021). Fusion protein EWS-FLI1 is incorporated into a protein granule in cells. RNA (New York, N.Y.), 27(8), 920-32.More infoEwing sarcoma is driven by fusion proteins containing a low complexity (LC) domain that is intrinsically disordered and a powerful transcriptional regulator. The most common fusion protein found in Ewing sarcoma, EWS-FLI1, takes its LC domain from the RNA-binding protein EWSR1 (Ewing Sarcoma RNA-binding protein 1) and a DNA-binding domain from the transcription factor FLI1 (Friend Leukemia Virus Integration 1). EWS-FLI1 can bind RNA polymerase II (RNA Pol II) and self-assemble through its low-complexity (LC) domain. The ability of RNA-binding proteins like EWSR1 to self-assemble or phase separate in cells has raised questions about the contribution of this process to EWS-FLI1 activity. We examined EWSR1 and EWS-FLI1 activity in Ewing sarcoma cells by siRNA-mediated knockdown and RNA-seq analysis. More transcripts were affected by the EWSR1 knockdown than expected and these included many EWS-FLI1 regulated genes. We reevaluated physical interactions between EWS-FLI1, EWSR1, and RNA Pol II, and employed a cross-linking based strategy to investigate protein assemblies associated with the proteins. The LC domain of EWS-FLI1 was required for the assemblies observed to form in cells. These results offer new insights into a protein assembly that may enable EWS-FLI1 to bind its wide network of protein partners and contribute to regulation of gene expression in Ewing sarcoma.
- Anderson, E. N., Morera, A. A., Kour, S., Cherry, J. D., Ramesh, N., Gleixner, A., Schwartz, J. C., Ebmeier, C., Old, W., Donnelly, C. J., Cheng, J. P., Kline, A. E., Kofler, J., Stein, T. D., & Pandey, U. B. (2021). Traumatic injury compromises nucleocytoplasmic transport and leads to TDP-43 pathology. eLife, 10.More infoTraumatic brain injury (TBI) is a predisposing factor for many neurodegenerative diseases, including amyotrophic lateral sclerosis (ALS), Alzheimer's disease (AD), Parkinson's disease (PD), and chronic traumatic encephalopathy (CTE). Although defects in nucleocytoplasmic transport (NCT) is reported ALS and other neurodegenerative diseases, whether defects in NCT occur in TBI remains unknown. We performed proteomic analysis on exposed to repeated TBI and identified resultant alterations in several novel molecular pathways. TBI upregulated nuclear pore complex (NPC) and nucleocytoplasmic transport (NCT) proteins as well as alter nucleoporin stability. Traumatic injury disrupted RanGAP1 and NPC protein distribution in flies and a rat model and led to coaggregation of NPC components and TDP-43. In addition, trauma-mediated NCT defects and lethality are rescued by nuclear export inhibitors. Importantly, genetic upregulation of nucleoporins in vivo and in vitro triggered TDP-43 cytoplasmic mislocalization, aggregation, and altered solubility and reduced motor function and lifespan of animals. We also found NUP62 pathology and elevated NUP62 concentrations in postmortem brain tissues of patients with mild or severe CTE as well as co-localization of NUP62 and TDP-43 in CTE. These findings indicate that TBI leads to NCT defects, which potentially mediate the TDP-43 pathology in CTE.
- Victor, R. A., Thompson, V. F., & Schwartz, J. C. (2021). Isolating and Analyzing Protein Containing Granules from Cells. Current protocols, 1(3), e35.More infoRecent advancements in detection methods have made protein condensates, also called granules, a major area of study, but tools to characterize these assemblies need continued development to keep up with evolving paradigms. We have optimized a protocol to separate condensates from cells using chemical cross-linking followed by size-exclusion chromatography (SEC). After SEC fractionation, the samples can be characterized by a variety of approaches including enzyme-linked immunosorbent assay, dynamic light scattering, electron microscopy, and mass spectrometry. The protocol described here has been optimized for cultured mammalian cells and E. coli expressing recombinant proteins. Since the lysates are fractionated by size, this protocol can be modified to study other large protein assemblies, including the nuclear pore complex, and for other tissues or organisms. © 2021 Wiley Periodicals LLC. Basic Protocol 1: SEC separation of cross-linked mammalian cell lysates Alternate Protocol: Preparation of non-crosslinked mammalian cells Basic Protocol 2: SEC separation of E. coli lysate Support Protocol 1: Detecting protein of interest by ELISA Support Protocol 2: TCA precipitation of SEC fractions.
- Kawaguchi, T., Rollins, M. G., Moinpour, M., Morera, A. A., Ebmeier, C. C., Old, W. M., & Schwartz, J. C. (2020). Changes to the TDP-43 and FUS Interactomes Induced by DNA Damage. Journal of proteome research, 19(1), 360-370.More infoThe RNA-binding proteins TDP-43 and FUS are tied as the third leading known genetic cause for amyotrophic lateral sclerosis (ALS), and TDP-43 proteopathies are found in nearly all ALS patients. Both the natural function and contribution to pathology for TDP-43 remain unclear. The intersection of functions between TDP-43 and FUS can focus attention for those natural functions mostly likely to be relevant to disease. Here, we compare the role played by TDP-43 and FUS, maintaining chromatin stability for dividing HEK293T cells. We also determine and compare the interactomes of TDP-43 and FUS, quantitating changes in those before and after DNA damage. Finally, selected interactions with known importance to DNA damage repair were validated by co-immunoprecipitation assays. This study uncovered TDP-43 and FUS binding to several factors important to DNA repair mechanisms that can be replication-dependent, -independent, or both. These results provide further evidence that TDP-43 has an important role in DNA stability and provide new ways that TDP-43 can bind to the machinery that guards DNA integrity in cells.
- Moinpour, M., & Schwartz, J. C. (2020). Determining protein structure with tyrosine bioconjugation. The FASEB Journal, 34(S1), 1-1. doi:10.1096/fasebj.2020.34.s1.06963More infoA hallmark of many neurodegenerative diseases like Alzheimer’s disease (AD), Amyotrophic Lateral Sclerosis (ALS) and Frontal Temporal Lobar Dementia (FTLD) is the formation of misfolded proteins into aggregates in the neuronal cells. These proteins often have intrinsically disordered regions which are enriched in a low complexity of amino acids such as Serine, Glycine, Glutamic acid and Tyrosine. Disordered proteins are challenging to study with common techniques such as x‐ray crystallography and NMR. Furthermore, common biorthogonal chemical reactions which mostly target lysine and cysteine residues cannot be used to determine the protein fold due to the low variety of amino acid composition in these proteins. However, a unique characteristic of several disordered proteins involved in neurodegenerative disease is their enrichment for tyrosines. Here we have developed an approach to assess tyrosine accessibility as a measure of levels in native vs misfolded forms of the proteins. To measure tyrosine accessibility we employed the ability of 4‐Phenyl‐1,2,4‐triazoline‐3,5‐dione (PTAD) to conjugate to tyrosine side‐chains. We assessed the targeted labeling of tyrosine’s for peptides, proteins, and cells. We have used this chemical approach to observe the transition of Bovine Serum Albumin (BSA), a well‐characterized and folded protein, to an unfolded state. We found that we could assess the local changes to protein structure by using LC‐MS/MS to quantify levels at each tyrosine position. We could confirm changes using fluorescent detection through click chemistry. Our long‐term goal is combining this approach and proteomics to quantify changes in cells to protein folding in comparable cellular states, such as disease and non‐diseased tissues. Support or Funding Information National Institute of Health, American Cancer Society
- Schwartz, J. C., Langlais, P. R., Jewett, J. C., Guzman, L. E., Barker, N. K., & Moinpour, M. (2020). Discriminating changes in protein structure using tyrosine conjugation. Protein Science.
- Krasnopolsky, S., Marom, L., Victor, R. A., Kuzmina, A., Schwartz, J. C., Fujinaga, K., & Taube, R. (2019). Fused in sarcoma silences HIV gene transcription and maintains viral latency through suppressing AFF4 gene activation. Retrovirology, 16(1), 16.More infoThe human immunodeficiency virus (HIV) cell reservoir is currently a main obstacle towards complete eradication of the virus. This infected pool is refractory to anti-viral therapy and harbors integrated proviruses that are transcriptionally repressed but replication competent. As transcription silencing is key for establishing the HIV reservoir, significant efforts have been made to understand the mechanism that regulate HIV gene transcription, and the role of the elongation machinery in promoting this step. However, while the role of the super elongation complex (SEC) in enhancing transcription activation of HIV is well established, the function of SEC in modulating viral latency is less defined and its cell partners are yet to be identified.
- Morera, A. A., Ahmed, N. S., & Schwartz, J. C. (2019). TDP-43 regulates transcription at protein-coding genes and Alu retrotransposons. Biochimica et biophysica acta. Gene regulatory mechanisms, 1862(10), 194434.More infoThe 43-kDa transactive response DNA-binding protein (TDP-43) is an example of an RNA-binding protein that regulates RNA metabolism at multiple levels from transcription and splicing to translation. Its role in post-transcriptional RNA processing has been a primary focus of recent research, but its role in regulating transcription has been studied for only a few human genes. We characterized the effects of TDP-43 on transcription genome-wide and found that TDP-43 broadly affects transcription of protein-coding and noncoding RNA genes. Among protein-coding genes, the effects of TDP-43 were greatest for genes
- Thompson, V. F., Victor, R. A., Morera, A. A., Moinpour, M., Liu, M. N., Kisiel, C. C., Pickrel, K., Springhower, C. E., & Schwartz, J. C. (2018). Transcription-Dependent Formation of Nuclear Granules Containing FUS and RNA Pol II. Biochemistry, 57(51), 7021-7032.More infoPurified recombinant FUsed in Sarcoma (FUS) assembles into an oligomeric state in an RNA-dependent manner to form large condensates. FUS condensates bind and concentrate the C-terminal domain of RNA polymerase II (RNA Pol II). We asked whether a granule in cells contained FUS and RNA Pol II as suggested by the binding of FUS condensates to the polymerase. We developed cross-linking protocols to recover protein particles containing FUS from cells and separated them by size exclusion chromatography. We found a significant fraction of RNA Pol II in large granules containing FUS with diameters of >50 nm or twice that of the RNA Pol II holoenzyme. Inhibition of transcription prevented the polymerase from associating with the granules. Altogether, we found physical evidence of granules containing FUS and RNA Pol II in cells that possess properties comparable to those of in vitro FUS condensates.
- Coyne, A. N., Lorenzini, I., Chou, C. C., Torvund, M., Rogers, R. S., Starr, A., Zaepfel, B. L., Levy, J., Johannesmeyer, J., Schwartz, J. C., Nishimune, H., Zinsmaier, K., Rossoll, W., Sattler, R., & Zarnescu, D. C. (2017). Post-transcriptional Inhibition of Hsc70-4/HSPA8 Expression Leads to Synaptic Vesicle Cycling Defects in Multiple Models of ALS. Cell reports, 21(1), 110-125.More infoAmyotrophic lateral sclerosis (ALS) is a synaptopathy accompanied by the presence of cytoplasmic aggregates containing TDP-43, an RNA-binding protein linked to ∼97% of ALS cases. Using a Drosophila model of ALS, we show that TDP-43 overexpression (OE) in motor neurons results in decreased expression of the Hsc70-4 chaperone at the neuromuscular junction (NMJ). Mechanistically, mutant TDP-43 sequesters hsc70-4 mRNA and impairs its translation. Expression of the Hsc70-4 ortholog, HSPA8, is also reduced in primary motor neurons and NMJs of mice expressing mutant TDP-43. Electrophysiology, imaging, and genetic interaction experiments reveal TDP-43-dependent defects in synaptic vesicle endocytosis. These deficits can be partially restored by OE of Hsc70-4, cysteine-string protein (Csp), or dynamin. This suggests that TDP-43 toxicity results in part from impaired activity of the synaptic CSP/Hsc70 chaperone complex impacting dynamin function. Finally, Hsc70-4/HSPA8 expression is also post-transcriptionally reduced in fly and human induced pluripotent stem cell (iPSC) C9orf72 models, suggesting a common disease pathomechanism.
- Ozdilek, B. A., Thompson, V. F., Ahmed, N. S., White, C. I., Batey, R. T., & Schwartz, J. C. (2017). Intrinsically disordered RGG/RG domains mediate degenerate specificity in RNA binding. Nucleic acids research, 45(13), 7984-7996.More infoRGG/RG domains are the second most common RNA binding domain in the human genome, yet their RNA-binding properties remain poorly understood. Here, we report a detailed analysis of the RNA binding characteristics of intrinsically disordered RGG/RG domains from Fused in Sarcoma (FUS), FMRP and hnRNPU. For FUS, previous studies defined RNA binding as mediated by its well-folded domains; however, we show that RGG/RG domains are the primary mediators of binding. RGG/RG domains coupled to adjacent folded domains can achieve affinities approaching that of full-length FUS. Analysis of RGG/RG domains from FUS, FMRP and hnRNPU against a spectrum of contrasting RNAs reveals that each display degenerate binding specificity, while still displaying different degrees of preference for RNA.
- Wang, X., Schwartz, J. C., & Cech, T. R. (2015). Nucleic acid-binding specificity of human FUS protein. Nucleic acids research, 43(15), 7535-43.More infoFUS, a nuclear RNA-binding protein, plays multiple roles in RNA processing. Five specific FUS-binding RNA sequence/structure motifs have been proposed, but their affinities for FUS have not been directly compared. Here we find that human FUS binds all these sequences with Kd (app) values spanning a 10-fold range. Furthermore, some RNAs that do not contain any of these motifs bind FUS with similar affinity. FUS binds RNA in a length-dependent manner, consistent with a substantial non-specific component to binding. Finally, investigation of FUS binding to different nucleic acids shows that it binds single-stranded DNA with three-fold lower affinity than ssRNA of the same length and sequence, while binding to double-stranded nucleic acids is weaker. We conclude that FUS has quite general nucleic acid-binding activity, with the various proposed RNA motifs being neither necessary for FUS binding nor sufficient to explain its diverse binding partners.
- Schwartz, J. C., Cech, T. R., & Parker, R. R. (2014). Biochemical Properties and Biological Functions of FET Proteins. Annual review of biochemistry.More infoMembers of the FET protein family, consisting of FUS, EWSR1, and TAF15, bind to RNA and contribute to the control of transcription, RNA processing, and the cytoplasmic fates of messenger RNAs in metazoa. FET proteins can also bind DNA, which may be important in transcription and DNA damage responses. FET proteins are of medical interest because chromosomal rearrangements of their genes promote various sarcomas and because point mutations in FUS or TAF15 can cause neurodegenerative diseases such as amyotrophic lateral sclerosis and frontotemporal lobar dementia. Recent results suggest that both the normal and pathological effects of FET proteins are modulated by low-complexity or prion-like domains, which can form higher-order assemblies with novel interaction properties. Herein, we review FET proteins with an emphasis on how the biochemical properties of FET proteins may relate to their biological functions and to pathogenesis. Expected final online publication date for the Annual Review of Biochemistry Volume 84 is June 02, 2015. Please see http://www.annualreviews.org/catalog/pubdates.aspx for revised estimates.