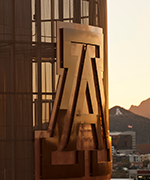
Laura Miller
- Professor, Mathematics
- Professor, Biomedical Engineering
- Member of the Graduate Faculty
- Professor, Applied Mathematics - GIDP
- (520) 621-6892
- Mathematics, Rm. 108
- Tucson, AZ 85721
- lauram9@arizona.edu
Biography
Laura Miller is a Professor of Mathematics and Biomedical Engineering at the University of Arizona. With nearly 30 years of experience in systems biomechanics, she employs experimental, computational, and theoretical methods to study organismal movement. Her research encompasses a variety of organisms, including insects, corals, and jellyfish. Notably, she has investigated the aerodynamics of insect flight, the fluid dynamics of jellyfish propulsion, and the flow patterns around corals. Her work has been recognized with the Burroughs Wellcome Career Award at the Scientific Interface and a National Science Foundation CAREER Award.
Beyond academia, Laura has been involved with horses since the age of 8, focusing on classical dressage, horse care, and equine facility management. She recently initiated an integrative research project on equine movement and respiration at the UA Al Marah Equine Center.
Degrees
- Ph.D. Mathematics
- New York University, New York, New York, United States
- M.S. Zoology
- Duke University, Durham, North Carolina, United States
- B.A. Biological Sciences
- University of Chicago, Chicago, Illinois, United States
Work Experience
- University of North Carolina, Chapel Hill, North Carolina (2007 - 2020)
Awards
- University of Arizona CURE Fellowship
- University of Arizona, Summer 2024
Interests
Research
My research focuses on the application of applied mathematics, mathematical biology, and fluid dynamics to understand the biomechanics of various organisms. I employ experimental, computational, and theoretical methods to study how organisms move and interact with their environments. This includes investigating the aerodynamics of insect flight, the fluid dynamics of jellyfish propulsion, and the flow patterns around corals. Additionally, I have initiated integrative research on equine movement and respiration at the UA Al Marah Equine Center.
Teaching
In the classroom, I am committed to mentoring students and fostering a collaborative research environment. I teach courses that bridge mathematics and biology, aiming to inspire innovation and contribute to the advancement of these fields. My goal is to equip students with the skills to apply mathematical and computational techniques to biological systems, preparing them for interdisciplinary research careers.
Courses
2025-26 Courses
-
Independent Study
APPL 599 (Fall 2025)
2024-25 Courses
-
Dissertation
APPL 920 (Spring 2025) -
Dissertation
APPL 920 (Fall 2024) -
Math modeling: bio fluid flow
MATH 481 (Fall 2024)
2023-24 Courses
-
Dissertation
MATH 920 (Spring 2024) -
Rsrch Ecology+Evolution
ECOL 610B (Spring 2024) -
Vector Calculus
MATH 223 (Spring 2024) -
Directed Research
MATH 492 (Fall 2023) -
Dissertation
MATH 920 (Fall 2023) -
Independent Study
APPL 599 (Fall 2023) -
Math modeling: bio fluid flow
MATH 481 (Fall 2023)
2022-23 Courses
-
Honors Independent Study
MATH 499H (Spring 2023) -
Honors Thesis
RNR 498H (Spring 2023) -
Research
MATH 900 (Spring 2023) -
Topics In Applied Math
MATH 577 (Spring 2023) -
Honors Independent Study
MATH 499H (Fall 2022) -
Honors Thesis
RNR 498H (Fall 2022) -
Independent Study
MATH 599 (Fall 2022) -
Math modeling: bio fluid flow
MATH 481 (Fall 2022) -
Methods Applied Mathematics I
APPL 581A (Fall 2022) -
Methods Applied Mathematics I
MATH 581A (Fall 2022)
2021-22 Courses
-
Honors Independent Study
MATH 499H (Spring 2022) -
Honors Independent Study
MATH 499H (Fall 2021) -
Independent Study
MATH 599 (Fall 2021) -
Math modeling: bio fluid flow
MATH 481 (Fall 2021)
2020-21 Courses
-
Honors Independent Study
MATH 499H (Spring 2021) -
Independent Study
MATH 499 (Spring 2021)
Scholarly Contributions
Books
- Miller, L. (2017).
Women in Mathematical Biology
. doi:10.1007/978-3-319-60304-9More infoAround the third week after gestation in embryonic development, the human heart consists only of a valvless tube, unlike a fully developed adult heart, which is multi-chambered. At this stage in development, the heart valves have not formed and so net flow of blood through the heart must be driven by a different mechanism. It is hypothesized that there are two possible mechanisms that drive blood flow at this stage - Liebau pumping (dynamic suction pumping or valveless pumping) and peristaltic pumping. We implement the immersed boundary method with adaptive mesh refinement (IBAMR) to numerically study the effect of hematocrit on the circulation around a valveless. Both peristalsis and dynamic suction pumping are considered. In the case of dynamic suction pumping, the heart and circulatory system is simplified as a flexible tube attached to a relatively rigid racetrack. For some Womersley number (Wo) regimes, there is significant net flow around the racetrack. We find that the addition of flexible blood cells does not significantly affect flow rates within the tube for Wo $\leq$ 10. On the other hand, peristalsis consistently drives blood around the racetrack for all Wo and for all hematocrit considered.
Chapters
- Miller, L. (2020).
Bumps, Ridges, and no Flows in Vein
. In The Art of Theoretical Biology. doi:10.1007/978-3-030-33471-0_53 - Strychalski, W., Bryant, S., Jadamba, B., Kilikian, E., Lai, X., Shahriyari, L., Segal, R., Wei, N., & Miller, L. (2018).
Fluid Dynamics of Nematocyst Prey Capture
. In Understanding Complex Biological Systems with Mathematics. Springer, Cham. doi:10.1007/978-3-319-98083-6_6More infoA nematocyst is a specialized organelle within cells of jellyfish and other Cnidarians that sting. Nematocysts are also present in some single-celled protists. They contain a barbed, venomous thread that accelerates faster than almost anything else in the animal kingdom. Here we simulate the fluid–structure interaction of the barbed thread accelerating through water to puncture its prey using the 2D immersed boundary method. For simplicity, our model describes the discharge of a single barb harpooning a single-celled organism, as in the case of dinoflagellates. One aspect of this project that is particularly interesting is that the micron-sized barbed thread reaches Reynolds numbers above one, where inertial effects become important. At this scale, even small changes in speed and shape can have dramatic effects on the local flow field. This suggests that the large variety of sizes and shapes of nematocysts may have important fluid dynamic consequences. We find that reaching the inertial regime is critical for hitting prey over short distances since the large boundary layers surrounding the barb characteristic of viscous dominated flows effectively push the prey out of the way. - Miller, L. (2017).
On the Dynamic Suction Pumping of Blood Cells in Tubular Hearts
. In Women in Mathematical Biology. doi:10.1007/978-3-319-60304-9_11More infoAround the third week after gestation in embryonic development, the human heart consists only of a valveless tube, unlike a fully developed adult heart, which is multi-chambered. At this stage in development, the heart valves have not formed and so net flow of blood through the heart must be driven by a different mechanism. It is hypothesized that there are two possible mechanisms that drive blood flow at this stage—Liebau pumping (dynamic suction pumping (DSP) or valveless pumping) and peristaltic pumping. We implement the immersed boundary method (IBM) with adaptive mesh refinement (IBAMR) to numerically study the effect of hematocrit on the circulation around a valveless tube. Both peristalsis and DSP are considered. In the case of DSP, the heart and circulatory system is simplified as a flexible tube attached to a relatively rigid racetrack. For some Womersley number (Wo) regimes, there is significant net flow around the racetrack. We find that the addition of flexible blood cells does not significantly affect flow rates within the tube forWo ≤ 10, except in the case forWo ≈ 1. 5 where we see a decrease in average flow with increasing volume fraction. On the other hand, peristalsis consistently drives blood around the racetrack for allWo and for all hematocrit considered. - Sheldon, K. S., Zhao, L., Chuang, A., Panayotova, I. N., Miller, L., & Bourouiba, L. (2017).
Revisiting the Physics of Spider Ballooning
. In Women in Mathematical Biology. Springer, Cham. doi:10.1007/978-3-319-60304-9_9More infoSpiders use a unique type of aerial dispersal called “ballooning” to move from one location to another. In order to balloon, a spider must first release one or more flexible, elastic, silk draglines from its spinnerets. Once enough force is generated on the dragline(s), the spider becomes airborne. This “take-off” stage of ballooning is followed by the “flight” stage and finally the “settling” stage when spiders land in a new location. Though the ecology of spider ballooning is well understood, little is known about the physical mechanisms. This is in part due to the significant challenge of describing the relevant physics for spiders that are ballooning across large distances. One difficulty, for example, is that properties of both the spider, such as body size and shape, and the silk dragline(s) can vary among species and individuals. In addition, the relevant physics may differ among the three stages of ballooning. Finally, models must take into account the interaction between the flexible dragline and air, and resolving this multi–scale, fluid–structure interaction can be particularly difficult. Here, we review the literature on spider ballooning, including the relevant physics, meteorological conditions that favor ballooning, and previous mechanical models used to describe ballooning. We then highlight challenges and open questions relevant to future modeling of spider ballooning. - Zhao, L., Panayotova, I. N., Chuang, A., Sheldon, K. S., Bourouiba, L., & Miller, L. (2017).
Flying Spiders: Simulating and Modeling the Dynamics of Ballooning
. In Women in Mathematical Biology. Springer, Cham. doi:10.1007/978-3-319-60304-9_10More infoSpiders use a type of aerial dispersal called “ballooning” to move from one location to another. In order to balloon, a spider releases a silk dragline from its spinnerets and when the movement of air relative to the dragline generates enough force, the spider takes flight. We have developed and implemented a model for spider ballooning to identify the crucial physical phenomena driving this unique mode of dispersal. Mathematically, the model is described as a fully coupled fluid–structure interaction problem of a flexible dragline moving through a viscous, incompressible fluid. The immersed boundary method has been used to solve this complex multi-scale problem. Specifically, we used an adaptive and distributed-memory parallel implementation of immersed boundary method (IBAMR). Based on the nondimensional numbers characterizing the surrounding flow, we represent the spider as a point mass attached to a massless, flexible dragline. In this paper, we explored three critical stages for ballooning, takeoff, flight, and settling in two dimensions. To explore flight and settling, we numerically simulate the spider in free fall in a quiescent flow. To model takeoff, we initially tether the spider-dragline system and then release it in two types of flows. Based on our simulations, we can conclude that the dynamics of ballooning is significantly influenced by the spider mass and the length of the dragline. Dragline properties such as the bending modulus also play important roles. While the spider-dragline is in flight, the instability of the atmosphere allows the spider to remain airborne for long periods of time. In other words, large dispersal distances are possible with appropriate wind conditions. - Hamlet, C., Miller, L., Rodriguez, T., & Santhanakrishnan, A. (2012).
The Fluid Dynamics of Feeding In the Upside-Down Jellyfish
. In Natural Locomotion in Fluids and on Surfaces. Springer, New York, NY. doi:10.1007/978-1-4614-3997-4_3More infoThe jellyfish has been the subject of numerous mathematical and physical studies ranging from the discovery of reentry phenomenon in electrophysiology to the development of axisymmetric methods for solving fluid-structure interaction problems. In the area of biologically inspired design, the jellyfish serves as a simple case study for understanding the fluid dynamics of unsteady propulsion with the goal of improving the design of underwater vehicles. In addition to locomotion, the study of jellyfish fluid dynamics could also lead to innovations in the design of filtration and sensing systems since an additional purpose of bell pulsations is to bring fluid to the organism for the purposes of feeding and nutrient exchange. The upside-down jellyfish, Cassiopea spp., is particularly well suited for feeding studies since it spends most of its time resting on the seafloor with its oral arms extended upward, pulsing to generate currents used for feeding and waste removal. In this paper, experimental measurements of the bulk flow fields generated by these organisms as well as the results from supporting numerical simulations are reviewed. Contraction, expansion, and pause times over the course of many contraction cycles are reported, and the effects of these parameters on the resulting fluid dynamics are explored. Of particular interest is the length of the rest period between the completion of bell expansion and the contraction of the next cycle. This component of the pulse cycle can be modeled as a Markov process. The discrete time Markov chain model can then be used to simulate cycle times using the distributions found empirically. Numerical simulations are used to explore the effects of the pulse characteristics on the fluid flow generated by the jellyfish. Preliminary results suggest that pause times have significant implications for the efficiency of particle capture and exchange.
Journals/Publications
- Moin, Z., Miller, L., & Battista, N. (2024). Ratcheting fluid pumps: Using generalized polynomial chaos expansions to assess pumping performance and sensitivity. . Physics of Fluids, 36(12), 121919.
- Santiago, M., & Miller, L. (2024). Interplay of Elasticity and Flow Velocity on Gorgonian Feeding and Implications for Bio-inspired Design. Ann NY Acad Sci, 15250.
- Miller, L. (2023). Multiscale flow between the branches and polyps of gorgonians. Journal of Experimental Biology, 226(5). doi:10.1242/jeb.244520More infoGorgonians, including sea fans, are soft corals well known for their elaborate branching structure and how they sway in the ocean. This branching structure can modify environmental flows to be beneficial for feeding in a particular range of velocities and, presumably, for a particular size of prey. As water moves through the elaborate branches, it is slowed, and recirculation zones can form downstream of the colony. At the smaller scale, individual polyps that emerge from the branches expand their tentacles, further slowing the flow. At the smallest scale, the tentacles are covered in tiny pinnules where exchange occurs. In this paper, we quantified the gap to diameter ratios for various gorgonians at the scale of the branches, the polyp tentacles and the pinnules. We then used computational fluid dynamics to determine the flow patterns at all three levels of branching. We quantified the leakiness between the branches, tentacles and pinnules over the biologically relevant range of Reynolds numbers and gap-to-diameter ratios, and found that the branches and tentacles can act as either leaky rakes or solid plates depending upon these dimensionless parameters. The pinnules, in contrast, mostly impede the flow. Using an agent-based modeling framework, we quantified plankton capture as a function of the gap-to-diameter ratio of the branches and the Reynolds number. We found that the capture rate depends critically on both morphology and Reynolds number. The results of the study have implications for how gorgonians modify ambient flows for efficient feeding and exchange.
- Miller, L., Hamlet, C., Strickland, C., & Nicolas, B. (2023). Multiscale flow between the branches and polyps of gorgonians. Journal of Experimental Biology, 226(5). doi:10.1242/jeb.244520
- Miller, L., Battista, N., Strickland, C., & Christina, H. (2022). Planktos: An agent-based modeling framework for computational fluid dynamics. Bulletin of Mathematical Biology, 84. doi:https://doi.org/10.1007/s11538-022-01027-1
- Miller, L., Santhanakrishnan, A., Battista, N., Hoover, A., Hamlet, C., & Gaddam, M. (2022). The presence of a substrate strengthens the jet generated by upside down jellyfish. Frontiers Marine Science, 9. doi:https://doi.org/10.3389/fmars.2022.847061
- Miller, L., Talkington, A., Davis, R., Datto, N., Goodwin, E., & Caron, K. (2022). Dermal lymphatic capillaries do not obey Murray's Law. Frontiers Cardiovascular Medicine, 12. doi:https://doi.org/10.3389/fcvm.2022.840305
- Santiago, M., Battista, N. A., Miller, L. A., & Khatri, S. (2022). Passive concentration dynamics incorporated into the library IB2d, a two-dimensional implementation of the immersed boundary method. Bioinspiration & biomimetics, 1748-3190.More infoIn this paper, we present an open-source software library that can be used to numerically simulate the advection and diffusion of a chemical concentration or heat density in a viscous fluid where a moving, elastic boundary drives the fluid and acts as a source or sink. The fully- coupled fluid-structure interaction problem of an elastic boundary in a viscous fluid is solved using Peskin's immersed boundary method. The addition or removal of the concentration or heat density from the boundary is solved using an immersed boundary-like approach in which the concentration is spread from the immersed boundary to the fluid using a regularized delta function. The concentration or density over time is then described by the advection-diffusion equation and numerically solved. This functionality has been added to our software library, IB2d, which provides an easy-to-use immersed boundary method in two dimensions with full implementations in MATLAB and Python. We provide four examples that illustrate the usefulness of the method. A simple rubber band that resists stretching and absorbs and releases a chemical concentration is simulated as a first example. Complete convergence results are presented for this benchmark case. Three more biological examples are presented: (1) an oscillating row of cylinders, representative of an idealized appendage used for filter-feeding or sniffing, (2) an oscillating plate in a background flow is considered to study the case of heat dissipation in a vibrating leaf, and (3) a simplified model of a pulsing soft coral where carbon dioxide is taken up and oxygen is released as a byproduct from the moving tentacles. This method is applicable to a broad range of problems in the life sciences, including chemical sensing by antennae, heat dissipation in plants and other structures, the advection-diffusion of morphogens during development, filter-feeding by marine organisms, and the release of waste products from organisms in flows.
- Hoover, A. P., Xu, N. W., Gemmell, B. J., Colin, S. P., Costello, J. H., Dabiri, J. O., & Miller, L. A. (2021). Neuromechanical wave resonance in jellyfish swimming. Proceedings of the National Academy of Sciences of the United States of America, 118(11).More infoFor organisms to have robust locomotion, their neuromuscular organization must adapt to constantly changing environments. In jellyfish, swimming robustness emerges when marginal pacemakers fire action potentials throughout the bell's motor nerve net, which signals the musculature to contract. The speed of the muscle activation wave is dictated by the passage times of the action potentials. However, passive elastic material properties also influence the emergent kinematics, with time scales independent of neuromuscular organization. In this multimodal study, we examine the interplay between these two time scales during turning. A three-dimensional computational fluid-structure interaction model of a jellyfish was developed to determine the resulting emergent kinematics, using bidirectional muscular activation waves to actuate the bell rim. Activation wave speeds near the material wave speed yielded successful turns, with a 76-fold difference in turning rate between the best and worst performers. Hyperextension of the margin occurred only at activation wave speeds near the material wave speed, suggesting resonance. This hyperextension resulted in a 34-fold asymmetry in the circulation of the vortex ring between the inside and outside of the turn. Experimental recording of the activation speed confirmed that jellyfish actuate within this range, and flow visualization using particle image velocimetry validated the corresponding fluid dynamics of the numerical model. This suggests that neuromechanical wave resonance plays an important role in the robustness of an organism's locomotory system and presents an undiscovered constraint on the evolution of flexible organisms. Understanding these dynamics is essential for developing actuators in soft body robotics and bioengineered pumps.
- Miller, L. A. (2021). Fluid flow through a deep-sea sponge could inspire engineering designs. Nature, 595(7868), 497-498.
- Miller, L. A., Hoover, A. P., Xu, N. W., Gemmell, B. J., Colin, S. P., Costello, J. H., & Dabiri, J. O. (2021). Neuromechanical wave resonance in jellyfish swimming. Proceedings of the National Academy of Sciences, 118(11). doi:10.1073/pnas.2020025118
- Senter, M., Ray, D., Strickland, C., Thomas, S., Talkington, A., & Miller, L. (2021). A Semi-Automated Finite Difference Mesh Creation Method for Use with Immersed Boundary Software Including IB2d and IBAMR. Biomimetics and Bioinspiration, 16.
- Battista, N. A., Douglas, D. R., Miller, L. A., Senter, D. M., Strickland, W. C., Strickland, C., Talkington, A. M., & Thomas, S. G. (2020). A semi-automated finite difference mesh creation method for use with immersed boundary software IB2d and IBAMR.. Bioinspiration & biomimetics, 16(1), 016008. doi:10.1088/1748-3190/ababb0More infoNumerous fluid-structure interaction problems in biology have been investigated using the immersed boundary method. The advantage of this method is that complex geometries, e.g., internal or external morphology, can easily be handled without the need to generate matching grids for both the fluid and the structure. Consequently, the difficulty of modeling the structure lies often in discretizing the boundary of the complex geometry (morphology). Both commercial and open source mesh generators for finite element methods have long been established; however, the traditional immersed boundary method is based on a finite difference discretization of the structure. Here we present a software library for obtaining finite difference discretizations of boundaries for direct use in the 2D immersed boundary method. This library provides tools for extracting such boundaries as discrete mesh points from digital images. We give several examples of how the method can be applied that include passing flow through the veins of insect wings, within lymphatic capillaries, and around starfish using open-source immersed boundary software.
- Hamlet, C., Strychalski, W., & Miller, L. (2020). Fluid Dynamics of Ballistic Strategies in Nematocyst Firing. Fluids. doi:https://doi.org/10.3390/fluids5010020
- Miller, L. A., Battista, N. A., Waldrop, L. D., He, Y., & Neary Peterman, T. (2020). Uncertainty quantification reveals the physical constraints on pumping by peristaltic hearts. Journal of The Royal Society Interface, 17(170), 20200232. doi:10.1098/rsif.2020.0232
- Ozalp, M. K., Miller, L. A., Dombrowski, T., Braye, M., Dix, T., Pongracz, L., Howell, R., Klotsa, D., Pasour, V., & Strickland, C. (2020). Experiments and Agent Based Models of Zooplankton Movement within Complex Flow Environments.. Biomimetics (Basel, Switzerland), 5(1), 2. doi:10.3390/biomimetics5010002More infoThe movement of plankton is often dictated by local flow patterns, particularly during storms and in environments with strong flows. Reefs, macrophyte beds, and other immersed structures can provide shelter against washout and drastically alter the distributions of plankton as these structures redirect and slow the flows through them. Advection-diffusion and agent-based models are often used to describe the movement of plankton within marine and fresh water environments and across multiple scales. Experimental validation of such models of plankton movement within complex flow environments is challenging because of the difference in both time and spatial scales. Organisms on the scale of 1 mm or less swim by beating their appendages on the order of 1 Hz and are advected meters to kilometers over days, weeks, and months. One approach to study this challenging multiscale problem is to insert actively moving agents within a background flow field. Open source tools to implement this sort of approach are, however, limited. In this paper, we combine experiments and computational fluid dynamics with a newly developed agent-based modeling platform to quantify plankton movement at the scale of tens of centimeters. We use Artemia spp., or brine shrimp, as a model organism given their availability and ease of culturing. The distribution of brine shrimp over time was recorded in a flow tank with simplified physical models of macrophytes. These simplified macrophyte models were 3D-printed arrays of cylinders of varying heights and densities. Artemia nauplii were injected within these arrays, and their distributions over time were recorded with video. The detailed three-dimensional flow fields were quantified using computational fluid dynamics and validated experimentally with particle image velocimetry. To better quantify plankton distributions, we developed an agent-based modeling framework, Planktos, to simulate the movement of plankton immersed within such flow fields. The spatially and temporally varying Artemia distributions were compared across models of varying heights and densities for both the experiments and the agent-based models. The results show that increasing the density of the macrophyte bed drastically increases the average time it takes the plankton to be swept downstream. The height of the macrophyte bed had less of an effect. These effects were easily observed in both experimental studies and in the agent-based simulations.
- Samson, J. E., & Miller, L. A. (2020). Collective Pulsing in Xeniid Corals: Part II-Using Computational Fluid Dynamics to Determine if There are Benefits to Coordinated Pulsing. Bulletin of mathematical biology, 82(6), 67.More infoCoordinated movements have been shown to enhance the speed or efficiency of swimming, flying, and pumping in many organisms. Coordinated pulsing has not been observed in many cnidarians (jellyfish, anemones, corals), as is the case for the xeniid corals considered in our corresponding paper. This observation opens the question as to whether xeniid corals, and cnidarians in general, do not coordinate their pulsing behavior for lack of a hydrodynamic advantage or for other reasons. For example, a diffuse nervous system with lack of substantial sensory input may not be capable of such coordination. Similarly, grouping may serve a defensive role rather than a fluid dynamic role. In this paper, the immersed boundary method is used to quantify the volumetric flux of fluid generated by an individual xeniid coral polyp in comparison with a pair of polyps. Both the distances between the polyps and the phase difference between each polyp are considered. More specifically, the fully coupled fluid-structure interaction problem of a coral polyp driving fluid flow is solved using a hybrid version of the immersed boundary method where the Navier-Stokes equations are solved using a finite differences and the elasticity equations describing the coral are solved using finite elements. We explore three possible hypotheses: (1) pulsing in pairs increases upward flow above the polyps and is thus beneficial, (2) these benefits vary with the polyps' pulsing phase difference, and (3) these benefits vary with the distance between the polyps. We find that there is no substantial hydrodynamic advantage to pulsing in a pair for any phase difference. The volumetric flux of fluid generated by each coral also decreases as the distance between polyps is decreased. This surprising result is consistent with measurements taken from another cnidarian with similar behavior, the upside down jellyfish, in which each medusa drives less flow when in a group.
- Samson, J. E., Ray, D. D., Porfiri, M., Miller, L. A., & Garnier, S. (2020). Collective Pulsing in Xeniid Corals: Part I-Using Computer Vision and Information Theory to Search for Coordination. Bulletin of mathematical biology, 82(7), 90.More infoXeniid corals (Cnidaria: Alcyonacea), a family of soft corals, include species displaying a characteristic pulsing behavior. This behavior has been shown to increase oxygen diffusion away from the coral tissue, resulting in higher photosynthetic rates from mutualistic symbionts. Maintaining such a pulsing behavior comes at a high energetic cost, and it has been proposed that coordinating the pulse of individual polyps within a colony might enhance the efficiency of fluid transport. In this paper, we test whether patterns of collective pulsing emerge in coral colonies and investigate possible interactions between polyps within a colony. We video recorded different colonies of Heteroxenia sp. in a laboratory environment. Our methodology is based on the systematic integration of a computer vision algorithm (ISOMAP) and an information-theoretic approach (transfer entropy), offering a vantage point to assess coordination in collective pulsing. Perhaps surprisingly, we did not detect any form of collective pulsing behavior in the colonies. Using artificial data sets, however, we do demonstrate that our methodology is capable of detecting even weak information transfer. The lack of a coordination is consistent with previous work on many cnidarians where coordination between actively pulsing polyps and medusa has not been observed. In our companion paper, we show that there is no fluid dynamic benefit of coordinated pulsing, supporting this result. The lack of coordination coupled with no obvious fluid dynamic benefit to grouping suggests that there may be non-fluid mechanical advantages to forming colonies, such as predator avoidance and defense.
- Waldrop, L., He, Y. Y., Battista, N., Neary, T., & Miller, L. (2020). Uncertainty quantification reveals the physical constraints on pumping by valveless, tubular hearts. Royal Society Interface. doi:doi:10.1098/rsif.2020.0232
- Bennett, A. E., Umbanhowar, J., Borrett, S. R., Apostol, K., Bever, J. D., Classen, A. T., Cuddington, K., Garrett, K. A., Gross, L., Hastings, A., Hoeksema, J. D., Hrynkiv, V., Karst, J., Kummel, M., Lee, C. T., Liang, C., Liao, W., Miller, L., Rojas, C., , Simms, E. L., et al. (2019). Beyond the black box: promoting mathematical collaborations for elucidating interactions in soil ecology. Ecosphere, 10(7). doi:10.1002/ecs2.2799More infoUnderstanding soil systems is critical because they form the structural and nutritional foundation for plants and thus every terrestrial habitat and agricultural system. In this paper, we encourage increased use of mathematical models to drive forward understanding of interactions in soil ecological systems. We discuss several distinctive features of soil ecosystems and empirical studies of them. We explore some perceptions that have previously deterred more extensive use of models in soil ecology and some advances that have already been made using models to elucidate soil ecological interactions. We provide examples where mathematical models have been used to test the plausibility of hypothesized mechanisms, to explore systems where experimental manipulations are currently impossible, or to determine the most important variables to measure in experimental and natural systems. To aid in the development of theory in this field, we present a table describing major soil ecology topics, the theory previously used, and providing key terms for theoretical approaches that could potentially address them. We then provide examples from the table that may either contribute to important incremental developments in soil science or potentially revolutionize our understanding of plant–soil systems. We challenge scientists and mathematicians to push theoretical explorations in soil systems further and highlight three major areas for the development of mathematical models in soil ecology: theory spanning scales and ecological hierarchies, processes, and evolution.
- Hobson, G., Miller, L., & Khatri, S. (2019). Dynamics of pulsing soft corals. Bulletin of the American Physical Society.
- Hoover, A. P., Porras, A. J., & Miller, L. A. (2019). Pump or coast: the role of resonance and passive energy recapture in medusan swimming performance. Journal of Fluid Mechanics, 863, 1031-1061. doi:10.1017/jfm.2018.1007More infoDiverse organisms that swim and fly in the inertial regime use the flapping or pumping of flexible appendages and cavities to propel themselves through a fluid. It has long been postulated that the speed and efficiency of locomotion are optimized by oscillating these appendages at their frequency of free vibration. In jellyfish swimming, a significant contribution to locomotory efficiency has been attributed to the effects passive energy recapture, whereby the bell is passively propelled through the fluid through its interaction with stopping vortex rings formed during each expansion of the bell. In this paper, we investigate the interplay between resonance and passive energy recapture using a three-dimensional implementation of the immersed boundary method to solve the fluid–structure interaction of an elastic oblate jellyfish bell propelling itself through a viscous fluid. The motion is generated through a fixed duration application of active tension to the bell margin, which mimics the action of the coronal swimming muscles. The pulsing frequency is then varied by altering the length of time between the application of applied tension. We find that the swimming speed is maximized when the bell is driven at its resonant frequency. However, the cost of transport is maximized by driving the bell at lower frequencies whereby the jellyfish passively coasts between active contractions through its interaction with the stopping vortex ring. Furthermore, the thrust generated by passive energy recapture was found to be dependent on the elastic properties of the jellyfish bell.
- Miller, L. (2019).
Fluid Dynamics of Ballistic Strategies in Nematocyst Firing
. Fluids, 5(1). doi:https://doi.org/10.3390/fluids5010020More infoNematocysts are stinging organelles used by members of the phylum Cnidaria (e.g., jellyfish, anemones, hydrozoans) for a variety of important functions including capturing prey and defense. Nematocysts are the fastest-known accelerating structures in the animal world. The small scale (microns) coupled with rapid acceleration (in excess of 5 million g) present significant challenges in imaging that prevent detailed descriptions of their kinematics. The immersed boundary method was used to numerically simulate the dynamics of a barb-like structure accelerating a short distance across Reynolds numbers ranging from 0.9–900 towards a passive elastic target in two dimensions. Results indicate that acceleration followed by coasting at lower Reynolds numbers is not sufficient for a nematocyst to reach its target. The nematocyst’s barb-like projectile requires high accelerations in order to transition to the inertial regime and overcome the viscous damping effects normally encountered at small cellular scales. The longer the barb is in the inertial regime, the higher the final velocity of the projectile when it touches its target. We find the size of the target prey does not dramatically affect the barb’s approach for large enough values of the Reynolds number, however longer barbs are able to accelerate a larger amount of surrounding fluid, which in turn allows the barb to remain in the inertial regime for a longer period of time. Since the final velocity is proportional to the force available for piercing the membrane of the prey, high accelerations that allow the system to persist in the inertial regime have implications for the nematocyst’s ability to puncture surfaces such as cellular membranes or even crustacean cuticle. - Miller, L. (2019).
Vortex Dynamics in Trabeculated Embryonic Ventricles
. J Cardiovasc Dev Dis, 6(1). doi:10.3390/jcdd6010006More infoProper heart morphogenesis requires a delicate balance between hemodynamic forces, myocardial activity, morphogen gradients, and epigenetic signaling, all of which are coupled with genetic regulatory networks. Recently both in vivo and in silico studies have tried to better understand hemodynamics at varying stages of veretebrate cardiogenesis. In particular, the intracardial hemodynamics during the onset of trabeculation is notably complex—the inertial and viscous fluid forces are approximately equal at this stage and small perturbations in morphology, scale, and steadiness of the flow can lead to significant changes in bulk flow structures, shear stress distributions, and chemical morphogen gradients. The immersed boundary method was used to numerically simulate fluid flow through simplified two-dimensional and stationary trabeculated ventricles of 72, 80, and 120 h post fertilization wild type zebrafish embryos and ErbB2-inhibited embryos at seven days post fertilization. A 2D idealized trabeculated ventricular model was also used to map the bifurcations in flow structure that occur as a result of the unsteadiness of flow, trabeculae height, and fluid scale ( R e ). Vortex formation occurred in intertrabecular regions for biologically relevant parameter spaces, wherein flow velocities increased. This indicates that trabecular morphology may alter intracardial flow patterns and hence ventricular shear stresses and morphogen gradients. A potential implication of this work is that the onset of vortical (disturbed) flows can upregulate Notch1 expression in endothelial cells in vivo and hence impacts chamber morphogenesis, valvulogenesis, and the formation of the trabeculae themselves. Our results also highlight the sensitivity of cardiac flow patterns to changes in morphology and blood rheology, motivating efforts to obtain spatially and temporally resolved chamber geometries and kinematics as well as the careful measurement of the embryonic blood rheology. The results also suggest that there may be significant changes in shear signalling due to morphological and mechanical variation across individuals and species. - Miller, L. (2019). Fluid dynamics of nutrient exchange through the branched arrays of sea fans and jellyfish oral arms. Bulletin of the American Physical Society.
- Miller, L. A., Hoover, A. P., & Davis, A. L. (2019). Lift and Drag Acting on the Shell of the American Horseshoe Crab (Limulus polyphemus). Bulletin of Mathematical Biology, 81(10), 3803-3822. doi:10.1007/s11538-019-00657-2More infoThe intertidal zone is a turbulent landscape where organisms face numerous mechanical challenges from powerful waves. A model for understanding the solutions to these physical problems, the American horseshoe crab (Limulus polyphemus), is a marine arthropod that mates in the intertidal zone, where it must contend with strong ambient flows to maintain its orientation during locomotion and reproduction. Possible strategies to maintain position include either negative lift generation or the minimization of positive lift in flow. To quantify flow over the shell and the forces generated, we laser-scanned the 3D shape of a horseshoe crab, and the resulting digital reconstruction was used to 3D-print a physical model. We then recorded the movement of tracking particles around the shell model with high-speed video and analyzed the time-lapse series using particle image velocimetry (PIV). The velocity vector fields from PIV were used to validate numerical simulations performed with the immersed boundary (IB) method. IB simulations allowed us to resolve the forces acting on the shell, as well as the local three-dimensional flow velocities and pressures. Both IB simulations and PIV analysis of vorticity and velocity at a flow speed of 13 cm/s show negative lift for negative and zero angles of attack, and positive lift for positive angles of attack in a free-stream environment. In shear flow simulations, we found near-zero lift for all orientations tested. Because horseshoe crabs are likely to be found primarily at near-zero angles of attack, we suggest that this negative lift helps maintain the orientation of the crab during locomotion and mating. This study provides a preliminary foundation for assessing the relationship between documented morphological variation and potential environmental variation for distinct populations of horseshoe crabs along the Atlantic Coast. It also motivates future studies which could consider the stability of the horseshoe crab in unsteady, oscillating flows.
- Samson, J. E., Miller, L. A., Holzman, R., Shavit, U., Khatri, S., & Ray, D. D. (2019). A novel mechanism of mixing by pulsing corals.. The Journal of experimental biology, 222(Pt 15). doi:10.1242/jeb.192518More infoThe dynamic pulsation of xeniid corals is one of the most fascinating phenomena observed in coral reefs. We quantify for the first time the flow near the tentacles of these soft corals, the active pulsations of which are thought to enhance their symbionts' photosynthetic rates by up to an order of magnitude. These polyps are approximately 1 cm in diameter and pulse at frequencies between approximately 0.5 and 1 Hz. As a result, the frequency-based Reynolds number calculated using the tentacle length and pulse frequency is on the order of 10 and rapidly decays as with distance from the polyp. This introduces the question of how these corals minimize the reversibility of the flow and bring in new volumes of fluid during each pulse. We estimate the Péclet number of the bulk flow generated by the coral as being on the order of 100-1000 whereas the flow between the bristles of the tentacles is on the order of 10. This illustrates the importance of advective transport in removing oxygen waste. Flow measurements using particle image velocimetry reveal that the individual polyps generate a jet of water with positive vertical velocities that do not go below 0.1 cm s-1 and with average volumetric flow rates of approximately 0.71 cm3 s-1 Our results show that there is nearly continual flow in the radial direction towards the polyp with only approximately 3.3% back flow. 3D numerical simulations uncover a region of slow mixing between the tentacles during expansion. We estimate that the average flow that moves through the bristles of the tentacles is approximately 0.03 cm s-1 The combination of nearly continual flow towards the polyp, slow mixing between the bristles, and the subsequent ejection of this fluid volume into an upward jet ensures the polyp continually samples new water with sufficient time for exchange to occur.
- Santiago, M., Miller, L., & Khatri, S. (2019). Chemical Interactions around Pulsing Soft Corals. Bulletin of the American Physical Society.
- Khatri, S., Alvarado, M., Miller, L., & Delgado, D. L. (2018). Numerical Simulations of Pulsating Soft Corals. Bulletin of the American Physical Society.
- Miller, L. (2018). Fluid dynamics of the flapping wings of the smallest insects. Bulletin of the American Physical Society.
- Ohdera, A. H., Abrams, M. J., Ames, C. L., Baker, D. M., Suescun-bolivar, L. P., Collins, A. G., Freeman, C. J., Gamero-mora, E., Goulet, T. L., Hofmann, D. K., Jaimes-becerra, A., Long, P. F., Marques, A. C., Miller, L. A., Mydlarz, L. D., Morandini, A. C., Newkirk, C. R., Putri, S. P., Samson, J. E., , Stampar, S. N., et al. (2018). Upside-Down but Headed in the Right Direction: Review of the Highly Versatile Cassiopea xamachana System. Frontiers in Ecology and Evolution, 6. doi:10.3389/fevo.2018.00035More infoThe upside-down jellyfish Cassiopea xamachana (Scyphozoa: Rhizostomeae) has been predominantly studied to understand its interaction with the endosymbiotic dinoflagellate algae Symbiodinium. As an easily culturable and tractable cnidarian model, it is an attractive alternative to stony corals to understanding the mechanisms driving establishment and maintenance of symbiosis. Cassiopea is also unique in requiring the symbiont in order to complete its transition to the adult stage, thereby providing an excellent model to understand symbiosis-driven development and evolution. Recently, the Cassiopea research system has gained interest beyond symbiosis in fields related to embryology, climate ecology, behavior, and more. With these developments, resources including genomes, transcriptomes, and laboratory protocols are steadily increasing. This review provides an overview of the broad range of interdisciplinary research that has utilized the Cassiopea model and highlights the advantages of using the model for future research. We dedicate this manuscript to Robert Trench, who inspired many of us to begin working in Cassiopea
- Santhanakrishnan, A., Jones, S., Miller, L., Peek, M. Y., Kasoju, V. T., Dickson, W. B., & Dickinson, M. H. (2018). Flow Structure and Force Generation on Flapping Wings at Low Reynolds Numbers Relevant to the Flight of Tiny Insects. Fluids, 3(3), 45. doi:10.3390/fluids3030045More infoIn contrast to larger species, little is known about the flight of the smallest flying insects, such as thrips and fairyflies. These tiny animals range from 300 to 1000 microns in length and fly at Reynolds numbers ranging from about 4 to 60. Previous work with numerical and physical models have shown that the aerodynamics of these diminutive insects is significantly different from that of larger animals, but most of these studies have relied on two-dimensional approximations. There can, however, be significant differences between two- and three-dimensional flows, as has been found for larger insects. To better understand the flight of the smallest insects, we have performed a systematic study of the forces and flow structures around a three-dimensional revolving elliptical wing. We used both a dynamically scaled physical model and a three-dimensional computational model at Reynolds numbers ranging from 1 to 130 and angles of attacks ranging from 0° to 90°. The results of the physical and computational models were in good agreement and showed that dimensionless drag, aerodynamic efficiency, and spanwise flow all decrease with decreasing Reynolds number. In addition, both the leading and trailing edge vortices remain attached to the wing over the scales relevant to the smallest flying insects. Overall, these observations suggest that there are drastic differences in the aerodynamics of flight at the scale of the smallest flying animals.
- Bucksch, A., Atta-boateng, A., Azihou, A. F., Battogtokh, D., Baumgartner, A., Binder, B. M., Braybrook, S. A., Coneva, V., Dewitt, T. J., Fletcher, A. G., Gehan, M. A., Diaz-martinez, D. H., Hong, L., Iyer-pascuzzi, A. S., Klein, L. L., Leiboff, S., Li, M., Lynch, J. P., Maizel, A., , Maloof, J. N., et al. (2017). Morphological Plant Modeling: Unleashing Geometric and Topological Potential within the Plant Sciences.. Frontiers in plant science, 8(900), 900. doi:10.3389/fpls.2017.00900More infoThe geometries and topologies of leaves, flowers, roots, shoots, and their arrangements have fascinated plant biologists and mathematicians alike. As such, plant morphology is inherently mathematical in that it describes plant form and architecture with geometrical and topological techniques. Gaining an understanding of how to modify plant morphology, through molecular biology and breeding, aided by a mathematical perspective, is critical to improving agriculture, and the monitoring of ecosystems is vital to modeling a future with fewer natural resources. In this white paper, we begin with an overview in quantifying the form of plants and mathematical models of patterning in plants. We then explore the fundamental challenges that remain unanswered concerning plant morphology, from the barriers preventing the prediction of phenotype from genotype to modeling the movement of leaves in air streams. We end with a discussion concerning the education of plant morphology synthesizing biological and mathematical approaches and ways to facilitate research advances through outreach, cross-disciplinary training, and open science. Unleashing the potential of geometric and topological approaches in the plant sciences promises to transform our understanding of both plants and mathematics.
- Hoover, A. P., Griffith, B. E., & Miller, L. A. (2017). Quantifying performance in the medusan mechanospace with an actively swimming three-dimensional jellyfish model. Journal of Fluid Mechanics, 813, 1112-1155. doi:10.1017/jfm.2017.3More infoIn many swimming and flying animals, propulsion emerges from the interplay of active muscle contraction, passive body elasticity and fluid–body interaction. Changes in the active and passive body properties can influence performance and cost of transport across a broad range of scales; they specifically affect the vortex generation that is crucial for effective swimming at higher Reynolds numbers. Theoretical models that account for both active contraction and passive elasticity are needed to understand how animals tune both their active and passive properties to move efficiently through fluids. This is particularly significant when one considers the phylogenetic constraints on the jellyfish mechanospace, such as the presence of relatively weak muscles that are only one cell layer thick. In this work, we develop an actively deforming model of a jellyfish immersed in a viscous fluid and use numerical simulations to study the role of active muscle contraction, passive body elasticity and fluid forces in the medusan mechanospace. By varying the strength of contraction and the flexibility of the bell margin, we quantify how these active and passive properties affect swimming speed and cost of transport. We find that for fixed bell elasticity, swimming speed increases with the strength of contraction. For fixed force of contractility, swimming speed increases as margin elasticity decreases. Varying the strength of activation in proportion to the elasticity of the bell margin yields similar swimming speeds, with a cost of transport is substantially reduced for more flexible margins. A scaling study reveals that performance declines as the Reynolds number decreases. Circulation analysis of the starting and stopping vortex rings showed that their strengths were dependent on the relative strength of activation with respect to the bell margin flexibility. This work yields a computational framework for developing a quantitative understanding of the roles of active and passive body properties in swimming.
- Miller, L. (2017).
IB2d Reloaded: A more powerful Python and MATLAB implementation of the immersed boundary method
. Mathematical Methods in the Applied Sciences. doi:10.1002/mma.4708 - Miller, L. (2017).
Bifurcations in valveless pumping techniques from a coupled fluid-structure-electrophysiology model in heart development
. Biomath, 6(2). doi:10.11145/j.biomath.2017.11.297More infoWe explore an embryonic heart model that couples electrophysiology and muscle-force generation to flow induced using a $2D$ fluid-structure interaction framework based on the immersed boundary method. The propagation of action potentials are coupled to muscular contraction and hence the overall pumping dynamics. In comparison to previous models, the electro-dynamical model does not use prescribed motion to initiate the pumping motion, but rather the pumping dynamics are fully coupled to an underlying electrophysiology model, governed by the FitzHugh-Nagumo equations. Perturbing the diffusion parameter in the FitzHugh-Nagumo model leads to a bifurcation in dynamics of action potential propagation. This bifurcation is able to capture a spectrum of different pumping regimes, with dynamic suction pumping and peristaltic-like pumping at the extremes. We find that more bulk flow is produced within the realm of peristaltic-like pumping. - Miller, L. (2017).
Fluid dynamics in heart development: effects of hematocrit and trabeculation
. Math Med Biol, 35(4), 493–516. doi:10.1093/imammb/dqx018 - Miller, L. (2017).
IB2d : a Python and MATLAB implementation of the immersed boundary method
. Bioinspir. Biomim., 12(3). doi:10.17615/cmcr-wt12 - Miller, L. (2017).
Pulsing corals: A story of scale and mixing
. Biomath. doi:10.11145/j.biomath.2017.12.169More infoEffective methods of fluid transport vary across scale. A commonly used dimensionless number for quantifying the effective scale of fluid transport is the Reynolds number, Re, which gives the ratio of inertial to viscous forces. What may work well for one Re regime may not produce significant flows for another. These differences in scale have implications for many organisms, ranging from the mechanics of how organisms move through their fluid environment to how hearts pump at various stages in development. Some organisms, such as soft pulsing corals, actively contract their tentacles to generate mixing currents that enhance photosynthesis. Their unique morphology and intermediate scale where both viscous and inertial forces are significant make them a unique model organism for understanding fluid mixing. In this paper, 3D fluid-structure interaction simulations of a pulsing soft coral are used to quantify fluid transport and fluid mixing across a wide range of Re. The results show that net transport is negligible for $Re - Miller, L. (2017).
Three-Dimensional Low Reynolds Number Flows near Biological Filtering and Protective Layers
. Fluids. doi:10.3390/fluids2040062More infoMesoscale filtering and protective layers are replete throughout the natural world. Within the body, arrays of extracellular proteins, microvilli, and cilia can act as both protective layers and mechanosensors. For example, blood flow profiles through the endothelial surface layer determine the amount of shear stress felt by the endothelial cells and may alter the rates at which molecules enter and exit the cells. Characterizing the flow profiles through such layers is therefore critical towards understanding the function of such arrays in cell signaling and molecular filtering. External filtering layers are also important to many animals and plants. Trichomes (the hairs or fine outgrowths on plants) can drastically alter both the average wind speed and profile near the leaf’s surface, affecting the rates of nutrient and heat exchange. In this paper, dynamically scaled physical models are used to study the flow profiles outside of arrays of cylinders that represent such filtering and protective layers. In addition, numerical simulations using the Immersed Boundary Method are used to resolve the three-dimensional flows within the layers. The experimental and computational results are compared to analytical results obtained by modeling the layer as a homogeneous porous medium with free flow above the layer. The experimental results show that the bulk flow is well described by simple analytical models. The numerical results show that the spatially averaged flow within the layer is well described by the Brinkman model. The numerical results also demonstrate, however, that the flow can be highly three-dimensional with fluid moving into and out of the layer. These effects are not described by the Brinkman model and may be significant for biologically relevant volume fractions. The results of this paper can be used to understand how variations in density and height of such structures can alter shear stresses and bulk flows. - Miller, L. A. (2017). Biomechanics: The aerodynamics buzz from mosquitoes.. Nature, 544(7648), 40-41. doi:10.1038/nature21904More infoMosquitoes flap their long, thin wings four times faster than similarly sized insects. Imaging and computational analysis of mosquito flight illuminates some aerodynamic mechanisms not seen before in animal flight. See Letter p.92 As anyone who has shared a bedroom with a mosquito will attest, mosquitos beat their wings fast enough for the irritating whine at about 800 Hz to be audible. Strangely, mosquito wings are long and thin, rather than the short and stubby wings expected to support rapid wing beats. The wing beat is also of rather low amplitude; the entire angular sweep of the wing is around 40 degrees, less than half that of the honey bee, whose 91 degree range is regarded as shallow. So how do mosquitoes fly at all? Bomphrey and colleagues show that in addition to generating lift by leading-edge vortices (as used by most insects) mosquitos employ trailing-edge vortices and a lift mechanism caused by the rotation of the wing. This adds to the expanding repertoire of mechanisms that insects use to stay airborne despite a seemingly unlikely airframe.
- Miller, L., Samson, J. E., & Khatri, S. (2017). Pulsing soft corals generate sustained upward jets and regions of strong mixing between their tentacles.. Bulletin of the American Physical Society.
- Santhanakrishnan, A., Senter, M., Armel, K. E., Miller, L., & Kasoju, V. T. (2017). Parachuting with bristled wings. Bulletin of the American Physical Society.
- Strickland, C., Kristensen, N. P., & Miller, L. (2017). Inferring stratified parasitoid dispersal mechanisms and parameters from coarse data using mathematical and Bayesian methods.. Journal of the Royal Society, Interface, 14(130), 20170005. doi:10.1098/rsif.2017.0005More infoBiological invasions have movement at the core of their success. However, due to difficulties in collecting data, medium- and long-distance dispersal of small insects has long been poorly understood and likely to be underestimated. The agricultural release of parasitic hymenoptera, a group of wasps that are critical for biological pest control, represents a rare opportunity to study the spread of insects on multiple spatial scales. As these insects are typically less than 1 mm in size and are challenging to track individually, a first-time biocontrol release will provide a known spatial position and time of initial release for all individuals that are subsequently collected. In this paper, we develop and validate a new mathematical model for parasitoid wasp dispersal from point release, as in the case of biocontrol. The model is derived from underlying stochastic processes but is fully deterministic and admits an analytical solution. Using a Bayesian framework, we then fit the model to an Australian dataset describing the multi-scale wind-borne dispersal pattern of Eretmocerus hayati Zolnerowich & Rose (Hymenoptera: Aphelinidae). Our results confirm that both local movements and long-distance wind dispersal are significant to the movement of parasitoids. The model results also suggest that low velocity winds are the primary indicator of dispersal direction on the field scale shortly after release, and that average wind data may be insufficient to resolve long-distance movement given inherent nonlinearities and heterogeneities in atmospheric flows. The results highlight the importance of collecting wind data when developing models to predict the spread of parasitoids and other tiny organisms.
- Strickland, C., Kristensen, N. P., & Miller, L. (2017). Modelling the Spread of Parasitoid Wasps from Point Release. Biomath Communications Supplement, 4(1).More infoParasitoid wasps are critical for biological pest control and are increasingly being used in agriculture to protect crops via release. However, due to their small size (often less than 1 mm), movement and long-distance dispersal of these wasps have long been poorly understood and likely underestimated. Recent data collected by Kristensen et al. [1] on the wind-borne dispersal pattern of E. hayati (0.7 mm long) provides a new and significant opportunity to develop a detailed, multi-scale model for the initial spread of small invasive insects and the dispersal of wind- or water-borne organisms in general. In this talk I will present a new model for parasitoid wasp dispersal from point release. The model is derived from underlying stochastic processes and, as a special case of the Fokker-Planck equation, is fully deterministic. The Python implementation of this model is general enough to be extended to other systems (e.g. plankton dispersal), and is capable of running month long simulations on the scale of 150 sq. km, while maintaining a resolution of 25x25 m - all within a minute on a common workstation. Speed is an essential component of our model because it permits fitting parameters to data using a Bayesian framework. Evaluation of the model in comparison with multi-scale, first-release data suggests the critical role of non-linear air flow for dispersal further than 1 km [2]. ...
- Wu, J., Cheng, Y., & Miller, L. A. (2017). An iterative source correction based immersed boundary-lattice Boltzmann method for thermal flow simulations. International Journal of Heat and Mass Transfer, 115, 450-460. doi:10.1016/j.ijheatmasstransfer.2017.08.004More infoAbstract Temperature jump at the boundary occurs when the conventional immersed boundary-lattice Boltzmann (IB-LB) method is applied to simulating the near boundary flows with heat transfer. To remedy this problem, an iterative correction is proposed to modify the heat source term in the IB-LB method. The source term in the LB equation is treated by Cheng’s scheme, in which the heat source at the next timestep is taken as unknowns and iteratively corrected until the resulting boundary temperature matches its desired value. Typical verification cases, including the two-dimensional (2D) heat transfer between two horizontal plates, the natural convection between two concentric circular cylinders, and 2D sedimentation of a single particle with heat convection are simulated to analyze the accuracy of the method. It is shown that the boundary temperature jump can be effectively removed for a certain range of LB relaxation time τ , while the first-order spatial convergence of the IB method is still maintained. Also, a theoretical analysis is conducted based on the case of heat transfer between two plates. It is shown that the proposed method outperforms the widely-used direct source method in treating the Dirichlet boundary conditions when τ is smaller than 1.624. To further demonstrate its capability for resolving complicated fluid-structure interaction problems, a three-dimensional sedimentation of a single particle in a vertical channel is analyzed. We find that the thermal convection may fundamentally affect the way the particle interacts with the surrounding fluid.
- Jones, S. K., Yun, Y. J., Hedrick, T. L., Griffith, B. E., & Miller, L. A. (2016). Bristles reduce the force required to 'fling' wings apart in the smallest insects.. The Journal of experimental biology, 219(Pt 23), 3759-3772. doi:10.1242/jeb.143362More infoThe smallest flying insects commonly possess wings with long bristles. Little quantitative information is available on the morphology of these bristles, and their functional importance remains a mystery. In this study, we (1) collected morphological data on the bristles of 23 species of Mymaridae by analyzing high-resolution photographs and (2) used the immersed boundary method to determine via numerical simulation whether bristled wings reduced the force required to fling the wings apart while still maintaining lift. The effects of Reynolds number, angle of attack, bristle spacing and wing-wing interactions were investigated. In the morphological study, we found that as the body length of Mymaridae decreases, the diameter and gap between bristles decreases and the percentage of the wing area covered by bristles increases. In the numerical study, we found that a bristled wing experiences less force than a solid wing. The decrease in force with increasing gap to diameter ratio is greater at higher angles of attack than at lower angles of attack, suggesting that bristled wings may act more like solid wings at lower angles of attack than they do at higher angles of attack. In wing-wing interactions, bristled wings significantly decrease the drag required to fling two wings apart compared with solid wings, especially at lower Reynolds numbers. These results support the idea that bristles may offer an aerodynamic benefit during clap and fling in tiny insects.
- Jones, S., Yun, Y., Hedrick, T. L., Griffith, B. E., & Miller, L. (2016). Bristles reduce force required to fling wings apart in small insects.. Bulletin of the American Physical Society.
- Khatri, S., Holzman, R., Miller, L., Samson, J. E., & Shavit, U. (2016). Pulsating Soft Corals. Bulletin of the American Physical Society.
- Miller, L. (2016).
Morphological plant modeling: Unleashing geometric and topological potential within the plant sciences
. Front. Plant Sci., 8. doi:10.1101/078832More infoAbstract Plant morphology is inherently mathematical in that morphology describes plant form and architecture with geometrical and topological descriptors. The geometries and topologies of leaves, flowers, roots, shoots and their spatial arrangements have fascinated plant biologists and mathematicians alike. Beyond providing aesthetic inspiration, quantifying plant morphology has become pressing in an era of climate change and a growing human population. Modifying plant morphology, through molecular biology and breeding, aided by a mathematical perspective, is critical to improving agriculture, and the monitoring of ecosystems with fewer natural resources. In this white paper, we begin with an overview of the mathematical models applied to quantify patterning in plants. We then explore fundamental challenges that remain unanswered concerning plant morphology, from the barriers preventing the prediction of phenotype from genotype to modeling the movement of leafs in air streams. We end with a discussion concerning the incorporation of plant morphology into educational programs. This strategy focuses on synthesizing biological and mathematical approaches and ways to facilitate research advances through outreach, cross-disciplinary training, and open science. This white paper arose from bringing mathematicians and biologists together at the National Institute for Mathematical and Biological Synthesis (NIMBioS) workshop titled “Morphological Plant Modeling: Unleashing Geometric and Topological Potential within the Plant Sciences” held at the University of Tennessee, Knoxville in September, 2015. Never has the need to quantify plant morphology been more imperative. Unleashing the potential of geometric and topological approaches in the plant sciences promises to transform our understanding of both plants and mathematics. - Miller, L. (2016). Passive aerial dispersal of insects and other arthropods. Bulletin of the American Physical Society.
- Miller, L., & Waldrop, L. D. (2016). Large-amplitude, short-wave peristalsis and its implications for transport.. Biomechanics and modeling in mechanobiology, 15(3), 629-42. doi:10.1007/s10237-015-0713-xMore infoValveless, tubular pumps are widespread in the animal kingdom, but the mechanism by which these pumps generate fluid flow is often in dispute. Where the pumping mechanism of many organs was once described as peristalsis, other mechanisms, such as dynamic suction pumping, have been suggested as possible alternative mechanisms. Peristalsis is often evaluated using criteria established in a technical definition for mechanical pumps, but this definition is based on a small-amplitude, long-wave approximation which biological pumps often violate. In this study, we use a direct numerical simulation of large-amplitude, short-wave peristalsis to investigate the relationships between fluid flow, compression frequency, compression wave speed, and tube occlusion. We also explore how the flows produced differ from the criteria outlined in the technical definition of peristalsis. We find that many of the technical criteria are violated by our model: Fluid flow speeds produced by peristalsis are greater than the speeds of the compression wave; fluid flow is pulsatile; and flow speed have a nonlinear relationship with compression frequency when compression wave speed is held constant. We suggest that the technical definition is inappropriate for evaluating peristalsis as a pumping mechanism for biological pumps because they too frequently violate the assumptions inherent in these criteria. Instead, we recommend that a simpler, more inclusive definition be used for assessing peristalsis as a pumping mechanism based on the presence of non-stationary compression sites that propagate unidirectionally along a tube without the need for a structurally fixed flow direction.
- Strickland, C., Pasour, V., & Miller, L. (2016). Low Reynolds number flow near tiny leaves, stems, and trichomes. Bulletin of the American Physical Society.
- Waldrop, L. D., Miller, L. A., & Khatri, S. (2016). A tale of two antennules: the performance of crab odour-capture organs in air and water.. Journal of the Royal Society, Interface, 13(125), 20160615. doi:10.1098/rsif.2016.0615More infoOdour capture is an important part of olfaction, where dissolved chemical cues (odours) are brought into contact with chemosensory structures. Antennule flicking by marine crabs is an example of discrete odour capture (sniffing) where an array of chemosensory hairs is waved through the water to create a flow-no flow pattern based on a narrow range of speeds, diameters of and spacings between hairs. Changing the speed of movement and spacing of hairs at this scale to manipulate flow represents a complicated fluid dynamics problem. In this study, we use numerical simulation of the advection and diffusion of a chemical gradient to reveal how morphological differences of the hair arrays affect odour capture. Specifically, we simulate odour capture by a marine crab (Callinectes sapidus) and a terrestrial crab (Coenobita rugosus) in both air and water to compare performance. We find that the antennule morphologies of each species are adaptions to capturing odours in their native habitats. Sniffing is an important part of odour capture for marine crabs in water where the diffusivity of odorant molecules is low and flow through the array is necessary. On the other hand, flow within the hair array diminishes odour-capture performance in air where diffusivities are high. This study highlights some of the adaptations necessary to transition from water to air.
- Baird, A., Miller, L., & Waldrop, L. D. (2015). Neuromechanical pumping: boundary flexibility and traveling depolarization waves drive flow within valveless, tubular hearts. Japan Journal of Industrial and Applied Mathematics, 32(3), 829-846. doi:10.1007/s13160-015-0195-3More infoIn this paper, we develop a neuromechanical model of pumping in a valveless, tubular heart inspired by the tunicate, Ciona savignyi. Valveless, tubular hearts are common throughout the animal kingdom. The vertebrate embryonic heart first forms as a valveless, tubular pump. The embryonic, juvenile, and adult hearts of many invertebrates are also valveless, tubular pumps. Several different pumping mechanisms have been propsed for tubular hearts, and it is not clear if all animals employ the same mechanism. We compare the flows generated by this pumping mechanisms to those produced by peristalsis using a prescribed contraction wave and to those produced by impedance pumping across a parameter space relevant to Ciona savignyi. The immersed boundary method is used to solve the fully-coupled fluid-structure interaction problem of an elastic tubular heart immersed in a viscous fluid. The FitzHugh–Nagumo equations are used to model the propagation of the action potential which initiates the contraction. We find that for the scales relevant to Ciona, both the neuromechanical pumping mechanism and peristalsis produce the strong flows observed in the tunicate heart. Only the neuromechanical model produces flow patterns with all of the characteristics reported for valveless, tubular hearts. Namely, the neuromechanical pump generates a bidirectional wave of contraction and peristalsis does not.
- Battista, N. A., Baird, A. J., & Miller, L. A. (2015). A Mathematical Model and MATLAB Code for Muscle-Fluid-Structure Simulations.. Integrative and comparative biology, 55(5), 901-11. doi:10.1093/icb/icv102More infoThis article provides models and code for numerically simulating muscle-fluid-structure interactions (FSIs). This work was presented as part of the symposium on Leading Students and Faculty to Quantitative Biology through Active Learning at the society-wide meeting of the Society for Integrative and Comparative Biology in 2015. Muscle mechanics and simple mathematical models to describe the forces generated by muscular contractions are introduced in most biomechanics and physiology courses. Often, however, the models are derived for simplifying cases such as isometric or isotonic contractions. In this article, we present a simple model of the force generated through active contraction of muscles. The muscles' forces are then used to drive the motion of flexible structures immersed in a viscous fluid. An example of an elastic band immersed in a fluid is first presented to illustrate a fully-coupled FSI in the absence of any external driving forces. In the second example, we present a valveless tube with model muscles that drive the contraction of the tube. We provide a brief overview of the numerical method used to generate these results. We also include as Supplementary Material a MATLAB code to generate these results. The code was written for flexibility so as to be easily modified to many other biological applications for educational purposes.
- Hedrick, T. L., Combes, S. A., & Miller, L. A. (2015). Recent developments in the study of insect flight1. Canadian Journal of Zoology, 93(12), 925-943. doi:10.1139/cjz-2013-0196More infoHere we review recent contributions to the study of insect flight, in particular those brought about by advances in experimental techniques. We focus particularly on the following areas: wing flexi...
- Hoover, A., & Miller, L. (2015). A numerical study of the benefits of driving jellyfish bells at their natural frequency.. Journal of theoretical biology, 374, 13-25. doi:10.1016/j.jtbi.2015.03.016More infoA current question in swimming and flight is whether or not driving flexible appendages at their resonant frequency results in faster or more efficient locomotion. It has been suggested that jellyfish swim faster when the bell is driven at its resonant frequency. The goal of this study was to determine whether or not driving a jellyfish bell at its resonant frequency results in a significant increase in swimming velocity. To address this question, the immersed boundary method was used to solve the fully coupled fluid structure interaction problem of a flexible bell in a viscous fluid. Free vibration numerical experiments were used to determine the resonant frequency of the jellyfish bell. The jellyfish bells were then driven at frequencies ranging from above and below the resonant frequency. We found that jellyfish do swim fastest for a given amount of applied force when the bells are driven near their resonant frequency. Nonlinear effects were observed for larger deformations, shifting the optimal frequency to higher than the resonant frequency. We also found that the benefit of resonant forcing decreases for lower Reynolds numbers.
- Hoover, A., Miller, L., & Griffith, B. E. (2015). Mechanical and scaling considerations for efficient jellyfish swimming. Bulletin of the American Physical Society.
- Jones, S. K., Laurenza, R., Hedrick, T. L., Griffith, B. E., & Miller, L. A. (2015). Lift vs. drag based mechanisms for vertical force production in the smallest flying insects.. Journal of theoretical biology, 384, 105-20. doi:10.1016/j.jtbi.2015.07.035More infoWe used computational fluid dynamics to determine whether lift- or drag-based mechanisms generate the most vertical force in the flight of the smallest insects. These insects fly at Re on the order of 4-60 where viscous effects are significant. Detailed quantitative data on the wing kinematics of the smallest insects is not available, and as a result both drag- and lift-based strategies have been suggested as the mechanisms by which these insects stay aloft. We used the immersed boundary method to solve the fully-coupled fluid-structure interaction problem of a flexible wing immersed in a two-dimensional viscous fluid to compare three idealized hovering kinematics: a drag-based stroke in the vertical plane, a lift-based stroke in the horizontal plane, and a hybrid stroke on a tilted plane. Our results suggest that at higher Re, a lift-based strategy produces more vertical force than a drag-based strategy. At the Re pertinent to small insect hovering, however, there is little difference in performance between the two strategies. A drag-based mechanism of flight could produce more vertical force than a lift-based mechanism for insects at Re
- Miller, L. (2015).
Large amplitude, short wave peristalsis and its implications for transport
. Biomechanics and Modeling in Mechanobiology. doi:10.7287/peerj.preprints.906v2More infoValveless, tubular pumps are widespread in the animal kingdom, but the mechanism by which these pumps generate fluid flow are often in dispute. Where the pumping mechanism of many organs was once described as peristalsis, other mechanisms, such as dynamic suction pumping, have been suggested as possible alternative mechanisms. Peristalsis is often evaluated using criteria established in a technical definition for mechanical pumps, but this definition is based on a small-amplitude, long-wave approximation which biological pumps often violate. In this study, we use a direct numerical simulation of large-amplitude, short-wave peristalsis to investigate the relationships between fluid flow, compression frequency, compression wave speed, and tube occlusion. We also explore how the flows produced differ from the criteria outlined in the technical definition of peristalsis. We find that many of the technical criteria are violated by our model: fluid flow speeds produced by peristalsis are greater than the speeds of the compression wave; fluid flow is pulsatile; and flow speed have a non-linear relationship with compression frequency when compression wave speed is held constant. We suggest that the technical definition is inappropriate for evaluating peristalsis as a pumping mechanism for biological pumps because they too frequently violate the assumptions inherent in these criteria. Instead, we recommend that a simpler, more inclusive definition be used for assessing peristalsis as a pumping mechanism based on the presence of non-stationary compression sites that propagate uni-directionally along a tube without the need for a structurally fixed flow direction. - Miller, L., Marzuola, J. L., & Hoover, A. (2015). The reconfiguration of broad leaves in strong winds and currents. Bulletin of the American Physical Society.
- Waldrop, L. D., & Miller, L. A. (2015). Introduction to the Symposium "Leading Students and Faculty to Quantitative Biology through Active Learning".. Integrative and comparative biology, 55(5), 898-900. doi:10.1093/icb/icv098More infoThe broad aim of this symposium and set of associated papers is to motivate the use of inquiry-based, active-learning teaching techniques in undergraduate quantitative biology courses. Practical information, resources, and ready-to-use classroom exercises relevant to physicists, mathematicians, biologists, and engineers are presented. These resources can be used to address the lack of preparation of college students in STEM fields entering the workforce by providing experience working on interdisciplinary and multidisciplinary problems in mathematical biology in a group setting. Such approaches can also indirectly help attract and retain under-represented students who benefit the most from "non-traditional" learning styles and strategies, including inquiry-based, collaborative, and active learning.
- Waldrop, L. D., & Miller, L. A. (2015). The role of the pericardium in the valveless, tubular heart of the tunicate Ciona savignyi.. The Journal of experimental biology, 218(Pt 17), 2753-63. doi:10.1242/jeb.116863More infoTunicates, small invertebrates within the phylum Chordata, possess a robust tubular heart which pumps blood through their open circulatory systems without the use of valves. This heart consists of two major components: the tubular myocardium, a flexible layer of myocardial cells that actively contracts to drive fluid down the length of the tube; and the pericardium, a stiff, outer layer of cells that surrounds the myocardium and creates a fluid-filled space between the myocardium and the pericardium. We investigated the role of the pericardium through in vivo manipulations on tunicate hearts and computational simulations of the myocardium and pericardium using the immersed boundary method. Experimental manipulations reveal that damage to the pericardium results in aneurysm-like bulging of the myocardium and major reductions in the net blood flow and percentage closure of the heart's lumen during contraction. In addition, varying the pericardium-to-myocardium (PM) diameter ratio by increasing damage severity was positively correlated with peak dye flow in the heart. Computational simulations mirror the results of varying the PM ratio experimentally. Reducing the stiffness of the myocardium in the simulations reduced mean blood flow only for simulations without a pericardium. These results indicate that the pericardium has the ability to functionally increase the stiffness of the myocardium and limit myocardial aneurysms. The pericardium's function is likely to enhance flow through the highly resistive circulatory system by acting as a support structure in the absence of connective tissue within the myocardium.
- Waldrop, L. D., Adolph, S. C., Behn, C. G., Braley, E., Drew, J. A., Full, R. J., Gross, L. J., Jungck, J. A., Kohler, B., Prairie, J. C., Shtylla, B., & Miller, L. A. (2015). Using Active Learning to Teach Concepts and Methods in Quantitative Biology.. Integrative and comparative biology, 55(5), 933-48. doi:10.1093/icb/icv097More infoThis article provides a summary of the ideas discussed at the 2015 Annual Meeting of the Society for Integrative and Comparative Biology society-wide symposium on Leading Students and Faculty to Quantitative Biology through Active Learning. It also includes a brief review of the recent advancements in incorporating active learning approaches into quantitative biology classrooms. We begin with an overview of recent literature that shows that active learning can improve students' outcomes in Science, Technology, Engineering and Math Education disciplines. We then discuss how this approach can be particularly useful when teaching topics in quantitative biology. Next, we describe some of the recent initiatives to develop hands-on activities in quantitative biology at both the graduate and the undergraduate levels. Throughout the article we provide resources for educators who wish to integrate active learning and technology into their classrooms.
- Hoover, A., Miller, L., & Griffith, B. E. (2014). Muscular Control of Turning and Maneuvering in Jellyfish Bells. Bulletin of the American Physical Society.
- Miller, L., & Hoover, A. (2014). Kinematics and Fluid Dynamics of Jellyfish Maneuvering. Bulletin of the American Physical Society.
- Santhanakrishnan, A., Robinson, A. K., Jones, S., Low, A. A., Gadi, S., Hedrick, T. L., Miller, L. A., & Lowe, A. (2014). Clap and fling mechanism with interacting porous wings in tiny insect flight.. The Journal of experimental biology, 217(Pt 21), 3898-909. doi:10.1242/jeb.084897More infoThe aerodynamics of flapping flight for the smallest insects such as thrips is often characterized by a 'clap and fling' of the wings at the end of the upstroke and the beginning of the downstroke. These insects fly at Reynolds numbers (Re) of the order of 10 or less where viscous effects are significant. Although this wing motion is known to augment the lift generated during flight, the drag required to fling the wings apart at this scale is an order of magnitude larger than the corresponding force acting on a single wing. As the opposing forces acting normal to each wing nearly cancel during the fling, these large forces do not have a clear aerodynamic benefit. If flight efficiency is defined as the ratio of lift to drag, the clap and fling motion dramatically reduces efficiency relative to the case of wings that do not aerodynamically interact. In this paper, the effect of a bristled wing characteristic of many of these insects was investigated using computational fluid dynamics. We performed 2D numerical simulations using a porous version of the immersed boundary method. Given the computational complexity involved in modeling flow through exact descriptions of bristled wings, the wing was modeled as a homogeneous porous layer as a first approximation. High-speed video recordings of free-flying thrips in take-off flight were captured in the laboratory, and an analysis of the wing kinematics was performed. This information was used for the estimation of input parameters for the simulations. Compared with a solid wing (without bristles), the results of the study show that the porous nature of the wings contributes largely to drag reduction across the Re range explored. The aerodynamic efficiency, calculated as the ratio of lift to drag coefficients, was larger for some porosities when compared with solid wings.
- Alben, S., Miller, L. A., & Peng, J. (2013). Efficient kinematics for jet-propelled swimming. Journal of Fluid Mechanics, 733, 100-133. doi:10.1017/jfm.2013.434More infoWe use computer simulations and an analytical model to study the relationship between kinematics and performance in jet-propelled jellyfish swimming. We prescribe different power-law kinematics for the bell contraction and expansion, and identify kinematics that yield high swimming speeds and/or high efficiency. In the simulations, high efficiency is found when the bell radius is a nearly linear function of time, and in a second case corresponding to ‘burst-and-coast’ kinematics. The analytical model studies the contraction phase only, and finds that the efficiency-optimizing bell radius as a function of time transitions from nearly linear (similar to the numerics) for small-to-moderate output power to exponentially decaying for large output power.
- Baird, A., & Miller, L. (2013). Electro-dynamic suction pumping at small scales. Bulletin of the American Physical Society.
- Battista, N. A., & Miller, L. (2013).
Pulsatile flow through idealized trabeculae
. Bulletin of the American Physical Society. - Hoover, A., & Miller, L. (2013). Do resonating bells increase jellyfish swimming performance. Bulletin of the American Physical Society.
- Jones, S., Laurenza, R., & Miller, L. (2013). A drag-based mechanism for vertical force production in the smallest flying insects. Bulletin of the American Physical Society.
- Miller, L. (2013). Reconfiguration of broad leaves into cones. Bulletin of the American Physical Society.
- Hamlet, C. L., & Miller, L. A. (2012). Feeding currents of the upside down jellyfish in the presence of background flow.. Bulletin of mathematical biology, 74(11), 2547-69. doi:10.1007/s11538-012-9765-6More infoThe upside-down jellyfish (Cassiopea spp.) is an ideal organism for examining feeding and exchange currents generated by bell pulsations due to its relatively sessile nature. Previous experiments and numerical simulations have shown that the oral arms play an important role in directing new fluid into the bell from along the substrate. All of this work, however, has considered the jellyfish in the absence of background flow, but the natural environments of Cassiopea and other cnidarians are dynamic. Flow velocities and directions fluctuate on multiple time scales, and mechanisms of particle capture may be fundamentally different in moving fluids. In this paper, the immersed boundary method is used to simulate a simplified jellyfish in flow. The elaborate oral arm structure is modeled as a homogenous porous layer. The results show that the oral arms trap vortices as they form during contraction and expansion of the bell. For constant flow conditions, the vortices are directed gently across the oral arms where particle capture occurs. For variable direction flows, the secondary structures change the overall pattern of the flow around the bell and appear to stabilize regions of mixing around the secondary mouths.
- Miller, L. (2012). Fluid dynamics of forward swimming and turning for jellyfish. Bulletin of the American Physical Society.
- Miller, L. A., & Alben, S. (2012). Interfacing mathematics and biology: a discussion on training, research, collaboration, and funding.. Integrative and comparative biology, 52(5), 616-21. doi:10.1093/icb/ics097More infoThis article summarizes the discussion at a workshop on "Working at the Interface of Mathematics and Biology" at the 2012 Annual Meeting of the Society for Integrative and Comparative Biology. The goal of this workshop was to foster an ongoing discussion by the community on how to effectively train students from the biological, physical, engineering, and mathematical sciences to work at the intersection of these fields. One major point of discussion centered on how to be a successful interdisciplinary researcher in terms of where to publish, how to successfully write grants, and how to navigate evaluations for tenure and promotion. An emphasis was placed on the importance of developing strong multidisciplinary collaborations and clearly defining one's career trajectory to the home discipline. Another focus of the discussion was on the training of students and postdoctoral fellows in interdisciplinary work and helping these junior researchers to launch their careers. The group emphasized the need for the development of publicly available resources for biologists to learn basic tools for mathematical modeling and for mathematicians and engineers to see how their fields may be applied to current topics in the life sciences.
- Miller, L. A., Goldman, D. I., Hedrick, T. L., Tytell, E. D., Wang, Z. J., Yen, J., & Alben, S. (2012). Using computational and mechanical models to study animal locomotion.. Integrative and comparative biology, 52(5), 553-75. doi:10.1093/icb/ics115More infoRecent advances in computational methods have made realistic large-scale simulations of animal locomotion possible. This has resulted in numerous mathematical and computational studies of animal movement through fluids and over substrates with the purpose of better understanding organisms' performance and improving the design of vehicles moving through air and water and on land. This work has also motivated the development of improved numerical methods and modeling techniques for animal locomotion that is characterized by the interactions of fluids, substrates, and structures. Despite the large body of recent work in this area, the application of mathematical and numerical methods to improve our understanding of organisms in the context of their environment and physiology has remained relatively unexplored. Nature has evolved a wide variety of fascinating mechanisms of locomotion that exploit the properties of complex materials and fluids, but only recently are the mathematical, computational, and robotic tools available to rigorously compare the relative advantages and disadvantages of different methods of locomotion in variable environments. Similarly, advances in computational physiology have only recently allowed investigators to explore how changes at the molecular, cellular, and tissue levels might lead to changes in performance at the organismal level. In this article, we highlight recent examples of how computational, mathematical, and experimental tools can be combined to ultimately answer the questions posed in one of the grand challenges in organismal biology: "Integrating living and physical systems."
- Miller, L. A., Santhanakrishnan, A., Jones, S., Hamlet, C., Mertens, K., & Zhu, L. (2012). Reconfiguration and the reduction of vortex-induced vibrations in broad leaves.. The Journal of experimental biology, 215(Pt 15), 2716-27. doi:10.1242/jeb.064501More infoFlexible plants, fungi and sessile animals reconfigure in wind and water to reduce the drag acting upon them. In strong winds and flood waters, for example, leaves roll up into cone shapes that reduce drag compared with rigid objects of similar surface area. Less understood is how a leaf attached to a flexible leaf stalk will roll up stably in an unsteady flow. Previous mathematical and physical models have only considered the case of a flexible sheet attached to a rigid tether in steady flow. In this paper, the dynamics of the flow around the leaf of the wild ginger Hexastylis arifolia and the wild violet Viola papilionacea are described using particle image velocimetry. The flows around the leaves are compared with those of simplified physical and numerical models of flexible sheets attached to both rigid and flexible beams. In the actual leaf, a stable recirculation zone is formed within the wake of the reconfigured cone. In the physical model, a similar recirculation zone is observed within sheets constructed to roll up into cones with both rigid and flexible tethers. Numerical simulations and experiments show that flexible rectangular sheets that reconfigure into U-shapes, however, are less stable when attached to flexible tethers. In these cases, larger forces and oscillations due to strong vortex shedding are measured. These results suggest that the three-dimensional cone structure in addition to flexibility is significant to both the reduction of vortex-induced vibrations and the forces experienced by the leaf.
- Santhanakrishnan, A., Dollinger, M., Hamlet, C. L., Colin, S. P., & Miller, L. A. (2012). Flow structure and transport characteristics of feeding and exchange currents generated by upside-down Cassiopea jellyfish.. The Journal of experimental biology, 215(Pt 14), 2369-81. doi:10.1242/jeb.053744More infoQuantifying the flows generated by the pulsations of jellyfish bells is crucial for understanding the mechanics and efficiency of their swimming and feeding. Recent experimental and theoretical work has focused on the dynamics of vortices in the wakes of swimming jellyfish with relatively simple oral arms and tentacles. The significance of bell pulsations for generating feeding currents through elaborate oral arms and the consequences for particle capture are not as well understood. To isolate the generation of feeding currents from swimming, the pulsing kinematics and fluid flow around the benthic jellyfish Cassiopea spp. were investigated using a combination of videography, digital particle image velocimetry and direct numerical simulation. During the rapid contraction phase of the bell, fluid is pulled into a starting vortex ring that translates through the oral arms with peak velocities that can be of the order of 10 cm s(-1). Strong shear flows are also generated across the top of the oral arms throughout the entire pulse cycle. A coherent train of vortex rings is not observed, unlike in the case of swimming oblate medusae such as Aurelia aurita. The phase-averaged flow generated by bell pulsations is similar to a vertical jet, with induced flow velocities averaged over the cycle of the order of 1-10 mm s(-1). This introduces a strong near-horizontal entrainment of the fluid along the substrate and towards the oral arms. Continual flow along the substrate towards the jellyfish is reproduced by numerical simulations that model the oral arms as a porous Brinkman layer of finite thickness. This two-dimensional numerical model does not, however, capture the far-field flow above the medusa, suggesting that either the three-dimensionality or the complex structure of the oral arms helps to direct flow towards the central axis and up and away from the animal.
- Hamlet, C., Santhanakrishnan, A., & Miller, L. A. (2011). A numerical study of the effects of bell pulsation dynamics and oral arms on the exchange currents generated by the upside-down jellyfish Cassiopea xamachana.. The Journal of experimental biology, 214(Pt 11), 1911-21. doi:10.1242/jeb.052506More infoMathematical and experimental studies of the flows generated by jellyfish have focused primarily on mechanisms of swimming. More recent work has also considered the fluid dynamics of feeding from currents generated during swimming. Here we capitalize on the benthic lifestyle of the upside-down jellyfish (Cassiopea xamachana) to explore the fluid dynamics of feeding uncoupled from swimming. A two-dimensional mathematical model is developed to capture the fundamental characteristics of the motion of the unique concave bell shape. Given the prominence of the oral arms, this structure is included and modeled as a porous layer that perturbs the flow generated by bell contractions. The immersed boundary method is used to solve the fluid-structure interaction problem. Velocity fields obtained from live organisms using digital particle image velocimetry were used to validate the numerical simulations. Parameter sweeps were used to numerically explore the effects of changes in pulse dynamics and the properties of the oral arms independently. Numerical experiments allow the opportunity to examine physical effects and limits within and beyond the biologically relevant range to develop a better understanding of the system. The presence of the prominent oral arm structures in the field of flow increased the flux of new fluid from along the substrate to the bell. The numerical simulations also showed that the presence of pauses between bell expansion and the next contraction alters the flow of the fluid over the bell and through the oral arms.
- Harenberg, S., Reis, J., & Miller, L. (2011). Simplified physical models of the flow around flexible insect wings at low Reynolds numbers. Bulletin of the American Physical Society, 64.
- Herschlag, G., & Miller, L. (2011). Reynolds number limits for jet propulsion: a numerical study of simplified jellyfish.. Journal of theoretical biology, 285(1), 84-95. doi:10.1016/j.jtbi.2011.05.035More infoThe Scallop theorem states that reciprocal methods of locomotion, such as jet propulsion or paddling, will not work in Stokes flow (Reynolds number=0). In nature the effective limit of jet propulsion is still in the range where inertial forces are significant. It appears that almost all animals that use jet propulsion swim at Reynolds numbers (Re) of about 5 or more. Juvenile squid and octopods hatch from the egg already swimming in this inertial regime. Juvenile jellyfish, or ephyrae, break off from polyps swimming at Re greater than 5. Many other organisms, such as scallops, rarely swim at Re less than 100. The limitations of jet propulsion at intermediate Re is explored here using the immersed boundary method to solve the 2D Navier-Stokes equations coupled to the motion of a simplified jellyfish. The contraction and expansion kinematics are prescribed, but the forward and backward swimming motions of the idealized jellyfish are emergent properties determined by the resulting fluid dynamics. Simulations are performed for both an oblate bell shape using a paddling mode of swimming and a prolate bell shape using jet propulsion. Average forward velocities and work put into the system are calculated for Re between 1 and 320. The results show that forward velocities rapidly decay with decreasing Re for all bell shapes when Re
- Miller, L. (2011). Uncovering the aerodynamics of the smallest insects using numerical and physical models. Bulletin of the American Physical Society, 64.
- Miller, L. A. (2011). Fluid dynamics of ventricular filling in the embryonic heart.. Cell biochemistry and biophysics, 61(1), 33-45. doi:10.1007/s12013-011-9157-9More infoThe vertebrate embryonic heart first forms as a valveless tube that pumps blood using waves of contraction. As the heart develops, the atrium and ventricle bulge out from the heart tube, and valves begin to form through the expansion of the endocardial cushions. As a result of changes in geometry, conduction velocities, and material properties of the heart wall, the fluid dynamics and resulting spatial patterns of shear stress and transmural pressure change dramatically. Recent work suggests that these transitions are significant because fluid forces acting on the cardiac walls, as well as the activity of myocardial cells that drive the flow, are necessary for correct chamber and valve morphogenesis. In this article, computational fluid dynamics was used to explore how spatial distributions of the normal forces acting on the heart wall change as the endocardial cushions grow and as the cardiac wall increases in stiffness. The immersed boundary method was used to simulate the fluid-moving boundary problem of the cardiac wall driving the motion of the blood in a simplified model of a two-dimensional heart. The normal forces acting on the heart walls increased during the period of one atrial contraction because inertial forces are negligible and the ventricular walls must be stretched during filling. Furthermore, the force required to fill the ventricle increased as the stiffness of the ventricular wall was increased. Increased endocardial cushion height also drastically increased the force necessary to contract the ventricle. Finally, flow in the moving boundary model was compared to flow through immobile rigid chambers, and the forces acting normal to the walls were substantially different.
- Santhanakrishnan, A., & Miller, L. A. (2011). Fluid dynamics of heart development.. Cell biochemistry and biophysics, 61(1), 1-22. doi:10.1007/s12013-011-9158-8More infoThe morphology, muscle mechanics, fluid dynamics, conduction properties, and molecular biology of the developing embryonic heart have received much attention in recent years due to the importance of both fluid and elastic forces in shaping the heart as well as the striking relationship between the heart's evolution and development. Although few studies have directly addressed the connection between fluid dynamics and heart development, a number of studies suggest that fluids may play a key role in morphogenic signaling. For example, fluid shear stress may trigger biochemical cascades within the endothelial cells of the developing heart that regulate chamber and valve morphogenesis. Myocardial activity generates forces on the intracardiac blood, creating pressure gradients across the cardiac wall. These pressures may also serve as epigenetic signals. In this article, the fluid dynamics of the early stages of heart development is reviewed. The relevant work in cardiac morphology, muscle mechanics, regulatory networks, and electrophysiology is also reviewed in the context of intracardial fluid dynamics.
- Zhu, L., He, G., Wang, S., Miller, L., Zhang, X., You, Q., & Fang, S. (2011). An immersed boundary method based on the lattice Boltzmann approach in three dimensions, with application. Computers & Mathematics With Applications, 61(12), 3506-3518. doi:10.1016/j.camwa.2010.03.022More infoThe immersed boundary (IB) method originated by Peskin has been popular in modeling and simulating problems involving the interaction of a flexible structure and a viscous incompressible fluid. The Navier-Stokes (N-S) equations in the IB method are usually solved using numerical methods such as FFT and projection methods. Here in our work, the N-S equations are solved by an alternative approach, the lattice Boltzmann method (LBM). Compared to many conventional N-S solvers, the LBM can be easier to implement and more convenient for modeling additional physics in a problem. This alternative approach adds extra versatility to the immersed boundary method. In this paper we discuss the use of a 3D lattice Boltzmann model (D3Q19) within the IB method. We use this hybrid approach to simulate a viscous flow past a flexible sheet tethered at its middle line in a 3D channel and determine a drag scaling law for the sheet. Our main conclusions are: (1) the hybrid method is convergent with first-order accuracy which is consistent with the immersed boundary method in general; (2) the drag of the flexible sheet appears to scale with the inflow speed which is in sharp contrast with the square law for a rigid body in a viscous flow.
- Santhanakrishnan, A., Miller, L., Hedrick, T. L., & Robinson, A. K. (2010). Clap and fling in tiny insects with porous wings. Bulletin of the American Physical Society, 63.
- Cooper, L., Fovargue, D., & Miller, L. (2009). Flow through flexible cylinders inspired by the endothelial glycocalyx. Bulletin of the American Physical Society, 62.
- Hamlet, C., & Miller, L. (2009). Numerical simulations of bell contractions of upside down jellyfish using the immersed boundary method. Bulletin of the American Physical Society, 62.
- Miller, L. A., & Peskin, C. S. (2009). Flexible clap and fling in tiny insect flight.. The Journal of experimental biology, 212(19), 3076-90. doi:10.1242/jeb.028662More infoOf the insects that have been filmed in flight, those that are 1 mm in length or less often clap their wings together at the end of each upstroke and fling them apart at the beginning of each downstroke. This ;clap and fling' motion is thought to augment the lift forces generated during flight. What has not been highlighted in previous work is that very large forces are required to clap the wings together and to fling the wings apart at the low Reynolds numbers relevant to these tiny insects. In this paper, we use the immersed boundary method to simulate clap and fling in rigid and flexible wings. We find that the drag forces generated during fling with rigid wings can be up to 10 times larger than what would be produced without the effects of wing-wing interaction. As the horizontal components of the forces generated during the end of the upstroke and beginning of the downstroke cancel as a result of the motion of the two wings, these forces cannot be used to generate thrust. As a result, clap and fling appears to be rather inefficient for the smallest flying insects. We also add flexibility to the wings and find that the maximum drag force generated during the fling can be reduced by about 50%. In some instances, the net lift forces generated are also improved relative to the rigid wing case.
- Miller, L., Santhanakrishnan, A., Hedrick, T. L., & Robinson, A. K. (2009). Flight of the smallest insects. Bulletin of the American Physical Society, 62.
- Santhanakrishnan, A., & Miller, L. (2009). An Experimental Investigation of the Feeding Currents Generated by Upside-Down Jellyfish. Bulletin of the American Physical Society, 62.
- Santhanakrishnan, A., Nguyen, N., Cox, J. G., & Miller, L. A. (2009). Flow within models of the vertebrate embryonic heart.. Journal of theoretical biology, 259(3), 449-61. doi:10.1016/j.jtbi.2009.04.020More infoVertebrate cardiogenesis is believed to be partially regulated by fluid forces imposed by blood flow in addition to myocardial activity and other epigenetic factors. To understand the flow field within the embryonic heart, numerical simulations using the immersed boundary method were performed on a series of models that represent simplified versions of some of the early morphological stages of heart development. The results of the numerical study were validated using flow visualization experiments conducted on equivalent dynamically scaled physical models. The chamber and cardiac cushion (or valve) depths in the models were varied, and Reynolds numbers ranging from 0.01 to 1000 corresponding to the scale of the early heart tube to the adult heart were considered. The observed results showed that vortex formation within the chambers occurred for Reynolds numbers on the order of 1-10. This transition to vertical flow appears to be highly sensitive to the chamber and cushion depths within the model. These fluid dynamic events could be important to induce shear sensing at the endothelial surface layer which is thought to be a part of regulating the proper morphological development and functionality of the valves.
- Gunderson, J., Santhanakrishnan, A., Nguyen, N., & Miller, L. (2008).
Comparison of Newtonian and Non-Newtonian Fluid Flow in Biological Models
. Bulletin of the American Physical Society, 61. - Leiderman, K. M., Miller, L. A., & Fogelson, A. L. (2008). The effects of spatial inhomogeneities on flow through the endothelial surface layer.. Journal of theoretical biology, 252(2), 313-25. doi:10.1016/j.jtbi.2008.01.013More infoFlow through the endothelial surface layer (the glycocalyx and adsorbed plasma proteins) plays an important but poorly understood role in cell signaling through a process known as mechanotransduction. Characterizing the flow rates and shear stresses throughout this layer is critical for understanding how flow-induced ionic currents, deformations of transmembrane proteins, and the convection of extracellular molecules signal biochemical events within the cell, including cytoskeletal rearrangements, gene activation, and the release of vasodilators. Previous mathematical models of flow through the endothelial surface layer are based upon the assumptions that the layer is of constant hydraulic permeability and constant height. These models also assume that the layer is continuous across the endothelium and that the layer extends into only a small portion of the vessel lumen. Results of these models predict that fluid shear stress is dissipated through the surface layer and is thus negligible near endothelial cell membranes. In this paper, such assumptions are removed, and the resultant flow rates and shear stresses through the layer are described. The endothelial surface layer is modeled as clumps of a Brinkman medium immersed in a Newtonian fluid. The width and spacing of each clump, hydraulic permeability, and fraction of the vessel lumen occupied by the layer are varied. The two-dimensional Navier-Stokes equations with an additional Brinkman resistance term are solved using a projection method. Several fluid shear stress transitions in which the stress at the membrane shifts from low to high values are described. These transitions could be significant to cell signaling since the endothelial surface layer is likely dynamic in its composition, density, and height.
- Nguyen, N., Santhanakrishnan, A., & Miller, L. (2008). Flow within Physical Models of the Vertebrate Embryonic Heart. Bulletin of the American Physical Society, 61.
- Santhanakrishnan, A., Miller, L., Dickson, W. B., & Dickinson, M. H. (2008). A Study of the Aerodynamics of Small Insect Flight. Bulletin of the American Physical Society, 61.
- Miller, L. (2005).
A computational fluid dynamics of `clap and fling' in the smallest insects
. Journal of Experimental Biology. doi:10.1242/jeb.01376More infoSUMMARY In this paper, we have used the immersed boundary method to solve the two-dimensional Navier–Stokes equations for two immersed wings performing an idealized `clap and fling' stroke and a `fling' half-stroke. We calculated lift coefficients as functions of time per wing for a range of Reynolds numbers (Re) between 8 and 128. We also calculated the instantaneous streamlines around each wing throughout the stroke cycle and related the changes in lift to the relative strength and position of the leading and trailing edge vortices. Our results show that lift generation per wing during the `clap and fling'of two wings when compared to the average lift produced by one wing with the same motion falls into two distinct patterns. For Re=64 and higher,lift is initially enhanced during the rotation of two wings when lift coefficients are compared to the case of one wing. Lift coefficients after fling and during the translational part of the stroke oscillate as the leading and trailing edge vortices are alternately shed. In addition, the lift coefficients are not substantially greater in the two-winged case than in the one-winged case. This differs from three-dimensional insect flight where the leading edge vortices remain attached to the wing throughout each half-stroke. For Re=32 and lower, lift coefficients per wing are also enhanced during wing rotation when compared to the case of one wing rotating with the same motion. Remarkably, lift coefficients following two-winged fling during the translational phase are also enhanced when compared to the one-winged case. Indeed, they begin about 70% higher than the one-winged case during pure translation. When averaged over the entire translational part of the stroke, lift coefficients per wing are 35% higher for the two-winged case during a 4.5 chord translation following fling. In addition, lift enhancement increases with decreasing Re. This result suggests that the Weis-Fogh mechanism of lift generation has greater benefit to insects flying at lower Re. Drag coefficients produced during fling are also substantially higher for the two-winged case than the one-winged case, particularly at lower Re. - Miller, L. A. (2005). Structural dynamics and resonance in plants with nonlinear stiffness.. Journal of theoretical biology, 234(4), 511-24. doi:10.1016/j.jtbi.2004.12.004More infoAlthough most biomaterials are characterized by strong stiffness nonlinearities, the majority of studies of plant biomechanics and structural dynamics focus on the linear elastic range of their behavior. In this paper, the effects of hardening (elastic modulus increases with strain) and softening (elastic modulus decreases with strain) nonlinearities on the structural dynamics of plant stems are investigated. A number of recent studies suggest that trees, crops, and other plants often uproot or snap when they are forced by gusting winds or waves at their natural frequency. This can be attributed to the fact that the deflections of the plant, and hence mechanical stresses along the stem and root system, are greatest during resonance. To better understand the effect of nonlinear stiffness on the resonant behavior of plants, plant stems have been modeled here as forced Duffing oscillators with softening or hardening nonlinearities. The results of this study suggest that the resonant behavior of plants with nonlinear stiffness is substantially different from that predicted by linear models of plant structural dynamics. Parameter values were considered over a range relevant to most plants. The maximum amplitudes of deflection of the plant stem were calculated numerically for forcing frequencies ranging from zero to twice the natural frequency. For hardening nonlinearities, the resonant behavior was 'pushed' to higher frequencies, and the maximum deflection amplitudes were lower than for the linear case. For softening nonlinearities, the resonant behavior was pushed to lower frequencies, and the maximum deflection amplitudes were higher than for the linear case. These nonlinearities could be beneficial or detrimental to the stability of the plant, depending on the environment. Damping had the effect of drastically decreasing deflection amplitudes and reducing the effect of the nonlinearities.
- Miller, L. (2004).
A computational study of flight in the smallest insects
. Journal of Experimental Biology.More infoIn order to study the aerodynamics of insect flight for Reynolds numbers in the range of 8–128, the Immersed Boundary Method was used to solve the two-dimensional incompressible Navier-Stokes equations with a flexible moving boundary. Although a number of experimental, numerical, and analytical studies have considered lift forces produced during flight in larger insects, only a few have considered Reynolds numbers below 100. To begin, the immersed boundary method was used to model two-dimensional stiff and flexible wings through one stroke cycle. Lift coefficients fell into two distinct patterns. For Reynolds numbers of 64 and higher, vortices were alternately shed from the wing forming the von Karman vortex street. For Reynolds numbers of 32 and below, leading and trailing edge vortices formed and remained ‘attached’ to the wing. The vortical symmetry produced at lower Reynolds numbers results in smaller lift forces and suggests that this transition is significant to flight in the smallest insects. In order to study wing-wing interactions, the immersed boundary method was used to model two rigid or flexible wings performing an idealized ‘clap and fling’ stroke. The results for stiff wings show that the lift generated during constant translation following ‘fling’ is substantially higher than the lift generated during steady translation. This effect becomes more significant with decreasing Reynolds number, and could explain why all tiny insects use the ‘clap and fling’. The results for flexible wings show that lift is also enhanced during translation following fling. In addition, the drag forces produced are significantly lower than those produced by a stiff wing using the same motion. - Miller, L. A., & Peskin, C. S. (2004). When vortices stick: an aerodynamic transition in tiny insect flight.. The Journal of experimental biology, 207(Pt 17), 3073-88. doi:10.1242/jeb.01138More infoWe have used computational fluid dynamics to study changes in lift generation and vortex dynamics for Reynolds numbers (Re) between 8 and 128. The immersed boundary method was used to model a two-dimensional wing through one stroke cycle. We calculated lift and drag coefficients as a function of time and related changes in lift to the shedding or attachment of the leading and trailing edge vortices. We find that the fluid dynamics around the wing fall into two distinct patterns. For Re> or =64, leading and trailing edge vortices are alternately shed behind the wing, forming the von Karman vortex street. For Re< or =32, the leading and trailing edge vortices remain attached to the wing during each 'half stroke'. In three-dimensional studies, large lift forces are produced by 'vortical asymmetry' when the leading edge vortex remains attached to the wing for the duration of each half stroke and the trailing edge vortex is shed. Our two-dimensional study suggests that this asymmetry is lost for Re below some critical value (between 32 and 64), resulting in lower lift forces. We suggest that this transition in fluid dynamics is significant for lift generation in tiny insects.
Proceedings Publications
- Stevens, T., Zhao, L., Zhang, W., Miller, L., & Courtney, R. J. (2019). Flying Spiders: Effects of the Dragline Length and the Spider Mass in Free-Fall. In Volume 1: Fluid Mechanics.More infoAbstract Many species of spiders move from one location to another using a remarkable aerial dispersal “ballooning”. By ballooning, spiders can reach distances as far as 3200 km and heights of up to 5 km. Though a large number of observations of spider ballooning have been reported, it remains a mysterious phenomenon due to the limited scientific observation of spider ballooning in the field, high uncertainties of the meteorological conditions and insufficient controlled laboratory experiments. Most of the ballooning spiders are spiderlings and spiders under 3 mm in length and 0.2 to 2 mg in mass with a few exceptions of large spiders (over 3 mm in length, over 5 mg in mass). What physical mechanism dominates the three stages of spider ballooning — take-off, flight, and settling? Many factors have been identified to influence the physical mechanism, including a spider’s mass, morphology, posture, the silken dragline properties, and local meteorological conditions (e.g., turbulence level, temperature and humidity). A thorough understanding of the roles of key parameters is not only of ecological significance but also critical to advanced bio-inspired technologies of airborne robotic devices. This work aims to determine how the dragline length and spider mass affect the interaction of the spider-dragline system in the free-fall scenario. Experiments using a thread of different lengths and a sphere of different masses to mimic the spider-dragline were carried out. The first sets of tests focused on the spider-dragline system, rather than the fluid flow. High-speed images of a spider-dragline falling in a closed container of air were recorded with 1500 frames per second at Reynolds numbers of several thousand, based on the spider dragline and the local relative velocity. Image data allow for tracking the vertical velocities and acceleration of the spider-dragline, as well as the drag force acting on the spider-dragline. Terminal velocities in the settling stage are compared with estimates using various fluid dynamics models in previous work. Such results under controlled laboratory conditions are expected to shed lights on the intriguing flow physics of spider ballooning at the settling stage and to inform future experiments and numerical models.
- Miller, L. (2017).
A fully coupled fluid-structure-muscle-electrophysiology model in heart development
. In Biomath.More infoThe vertebrate heart begins to pump when its morphology is nothing more than a valveless tube, composed of an outer layer of myocardial cells surrounding an inner layer of endocardial cells. It has been proposed that the purpose of the embyronic heartbeat is to aid in the growth and shaping of the heart itself in organogenesis, rather than the delivery of oxygen and nutrients [1]. These heart tubes have been described as peristaltic and impedance pumps. Impedance pumping assumes a single actuation point of contraction, while traditional peristalsis assumes a traveling wave of actuation. In addition to differences in flow, this inherently implies differences in the conduction system. It is possible to transition from one pumping mechanism to the other with a change in the diffusivity of the action potential. Using an open source implementation of the immersed boundary method, \textit{IB2D} [2], we developed a fully coupled fluid-structure-muscle-electrophysiology model of the embryonic heart. We find that differences in the resulting pumping behavior, greatly affects the advection and diffusion of a chemical gradient within the heart tube. These chemical gradients, e.g., morphogens, could serve as an essential epigenetic signal required for proper cardiogenesis [3] - Miller, L. (2017).
Feeding and exchange flows produced by soft corals and upside down jellyfish
. In Biomath.More infoUnderstanding how the morphology of soft marine invertebrates affects the hydrodynamics of feeding and nutrient exchange is important for understanding this diversity of life and predicting how climate change will alter the distributions of these organisms. Soft coral of the family Xeniidae pulse with their tentacles to enhance the photosynthetic rates of symbiotic zooxanthellae. Bell pulsations of the upside down jellyfish \textit{Cassiopea spp.} create feeding currents and may also enhance photosynthesis. Other soft invertebrates use active movements for efficient low speed cruising or alternatively for quick swimming bursts. In this presentation, we use videography to quantify the movement of soft corals and upside down jellyfish. We also use particle image velocimetry to reveal the flow fields these movements create. Numerical simulations of simplified models of jellyfish and soft corals are used then to quantify and compare exchange mechanisms associated with pulsations. Numerical models allow us to isolate fundamental effects of different parameters as well as to extend the examination beyond experimental feasibility or even biological possibilities. In this way computational studies present an opportunity to explore fundamental physical limits on biological mechanisms. - Miller, L. (2017).
Under the sea: Pulsing corals in ambient flow
. In Mathematical Methods and Models in Biosciences.More infoWhile many organisms filter feed and exchange heat or nutrients in flow, few benthic organisms also actively pulse to enhance feeding and exchange. One example is the pulsing soft coral (Heteroxenia fuscescens). Pulsing corals live in colonies, where each polyp actively pulses through contraction and relaxation of their tentacles. The pulses are typically out of phase and without a clear pattern. These corals live in lagoons and bays found in the Red Sea and Indian Ocean where they at times experience strong ambient flows. In this paper, $3D$ fluid-structure interaction simulations are used to quantify the effects of ambient flow on the exchange currents produced by the active contraction of pulsing corals. We find a complex interaction between the flows produced by the coral and the background flow. The dynamics can either enhance or reduce the upward jet generated in a quiescent medium. The pulsing behavior also slows the average horizontal flow near the polyp when there is a strong background flow. The dynamics of these flows have implications for particle capture and nutrient exchange. - Miller, L. (2013).
Broad Leaves in Strong Flow
. In American Physical Society Division of Fluid Dynamics.More infoFlexible broad leaves are thought to reconfigure in the wind and water to reduce the drag forces that act upon them. Simple mathematical models of a flexible beam immersed in a two-dimensional flow will also exhibit this behavior. What is less understood is how the mechanical properties of a leaf in a three-dimensional flow will passively allow roll up into a cone shape and reduce both drag and vortex induced oscillations. In this fluid dynamics video, the flows around the leaves are compared with those of simplified sheets using 3D numerical simulations and physical models. For some reconfiguration shapes, large forces and oscillations due to strong vortex shedding are produced. In the actual leaf, a stable recirculation zone is formed within the wake of the reconfigured cone. In physical and numerical models that reconfigure into cones, a similar recirculation zone is observed with both rigid and flexible tethers. These results suggest that the three-dimensional cone structure in addition to flexibility is significant to both the reduction of vortex-induced vibrations and the forces experienced by the leaf. - Overman, R. E., Overman, R. E., Prins, J. F., Prins, J. F., Miller, L. A., Miller, L. A., Minion, M. L., & Minion, M. L. (2013). Dynamic Load Balancing of the Adaptive Fast Multipole Method in Heterogeneous Systems. In 2013 IEEE International Symposium on Parallel & Distributed Processing, Workshops and Phd Forum, 1126-1135.More infoSimulations of colliding galaxies or fluid dynamics at immersed flexible boundaries are most accurately and efficiently accomplished using the adaptive fast multipole method (AFMM) to solve an underlying n-body problem whose localized density varies with the time-dependent evolution of the system under study. Parallelization of the AFMM presents a challenging load balancing problem that must be addressed dynamically as the system evolves. We consider parallelization of the AFMM for time dependent problems using a heterogeneous shared memory compute node consisting of multi-core processors and GPU accelerators. OpenMP task parallelism is used within the CPU cores to parallelize the construction and maintenance of the adaptive spatial decomposition tree and its traversal to compute far-field interactions at each leaf node in the tree. Concurrently, GPUs evaluate all near-field interactions using all-pairs computations. In addition to accurately resolving many physical phenomena out of reach using the uniform FMM, the more complex AFMM permits the number of bodies in leaf cells to be globally and locally varied in order to minimize the CPU and GPU time. We present a cost model and incremental adjustment strategy to load balance the AFMM on a heterogeneous system. We demonstrate using these techniques that a simulation can maintain load balance over hundreds of time steps on a heterogeneous system with 10 CPU cores and 4 GPUs with less than 2% overhead, while achieving a 98X speedup over a serial computation using a single CPU core.
- Miller, L. (2010).
Feeding Currents Generated by Upside Down Jellyfish
. In American Physical Society Division of Fluid Dynamics.More infoWe present fluid dynamics videos of the pulsing dynamics and the resulting fluid flow generated by the upside down jellyfish, Cassiopea spp. Medusae of this genus are unusual in that they typically rest upside down on the ocean floor and pulse their bells to generate feeding currents, only swimming when significantly disturbed. The pulsing kinematics and fluid flow around these upside down jellyfish is investigated using a combination of videography, flow visualization, and numerical simulation. Significant mixing occurs around and directly above the oral arms and secondary mouths. Numerical simulations using the immersed boundary method with a porous layer representing the oral arms agree with the experimental results. The simulations also suggest that the presence of porous oral arms induce net horizontal flow towards the bell. Coherent vortex rings are not seen in the wake above the jellyfish, but starting and stopping vortices are observed before breaking up as they pass through the elaborate oral arms (if extended). - Miller, L. (2008).
Leaf roll-up and aquaplaning in strong winds and floods
. In American Physical Society Division of Fluid Dynamics.More infoFlexible plants, fungi, and sessile animals are thought to reconfigure in the wind and water to reduce the drag forces that act upon them. In strong winds, for example, leaves roll up into cone shapes that reduce flutter and drag when compared to paper cut-outs with similar shapes and flexibility. During flash floods, herbaceous broad leaves aquaplane on the surface of the water which reduces drag. Simple mathematical models of a flexible beam immersed in a two-dimensional flow will also reconfigure in flow. What is less understood is how the mechanical properties of a two-dimensional leaf in a three-dimensional flow will passively allow roll up and aquaplaning. In this study, we film leaf roll-up and aquaplaning in tree and vine leaves in both strong winds and water flows.
Presentations
- Miller, L. (2025, January). Equine biomechanics. ATCO SpaceLab Speaker Series. Online: ATCO.
- Miller, L. (2025, January). Quantifying and mathematical modeling gait asymmetries in horses. . The Society for Integrative and Comparative Biology Annual Meeting. Atlanta, GA: Society for Integrative and Comparative Biology.
- Miller, L. (2023). Flows through soft corals and other cnidarians. . Mathematical and Computational Biology, ICERM. Providence, RI.
- Miller, L. (2024, June). Flows generated by jellyfish with prominent oral arms. SIAM Conference on the Life Sciences (LS24). Portland, OR: Society for Industrial and Applied Mathematics.
- Miller, L. (2024, November). Flows around some species of soft corals. ISAAC-ICMAM Conference of Women in Mathematics. Online: ISAAC-ICMAM.
- Miller, L. (2024, October). Tutorial: Using computational fluid dynamics to understand marine systems. . AIMEC-NSF Joint Workshop for the Model Species Cassiopea: Molecular Analysis with Gridion and Fluidic Dynamics. Tohoku University’s Onagawa Field Center, Japan.: AIMEC-NSF.
- Miller, L., & Khatri, S. (2024, June). Computational fluid dynamics tutorial . Microscale Ocean Biophysics Meeting. Heron Island, Australia: Microscale Ocean Biophysics.
- Miller, L. (2023). Couple Fluid-Structure Interaction Simulations of the Jets Generated by Upside-Down Jellyfish. Coupled Problems 2023. Chania, Crete, Greece.
- Miller, L. (2023). Flows around some soft corals. 2023 AMS Fall Southeastern Sectional Meeting. Mobile, AL.
- Miller, L. (2023). Flows around some soft corals. 2nd SIAM Northern States Section Conference. Logan, UT.
- Miller, L. (2022). Multiscale flow through the branches of gorgonians. Microscale Ocean Biophysics Meeting. Mallorca, Spain.
- Miller, L. (2022). Using computational fluid dynamics to understand muscle driven movement: Case students in jellyfish and corals. Applied and Interdisciplinary Mathematics Seminar, University of Michigan.
- Miller, L. (2022). Using computational fluid dynamics to understand the mechanics of jellyfish swimming and feeding. The BPPB Seminar (online).
- Miller, L. (2022). Electromechanical Pumping in Embryonic Heart Tubes. SIAM Conference on the Life Sciences.
- Miller, L. (2022). Multiscale flow between the branches and polyps of gorgonians.. The 2022 Annual Meeting of the Society of Integrative and Comparative Biology (SICB+). Phoenix, AZ: Society of Integrative and Comparative Biology.
- Miller, L., & Aghav, H. (2022). Effects of Wing Bristles and Wing Flexibility on the Aerodynamics of the Smallest Flying Insects.. SIAM Annual Meeting. Pittsburgh, PA.
- Miller, L., & Hu, D. (2022). Fluid-Organism Interactions: Mathematical and Numerical Approaches. UCI Center for Integrative Movement Sciences (online).
- Miller, L., Glickenstein, D. A., & Lin, K. (2022). Applied Mathematics and Statistics for Data-Driven Discovery. SIAM Conference on Applied Mathematics Education.
- Santiago, M. K., Miller, L., Battista, N., & Khatri, S. (2022, March). Using IB2d to simulate chemical concentrations and heat transfer around marine organisms. Ocean Science Meeting. Online.
- Khatri, S., Hobson, G., Mitchell, K. A., Miller, L., & Santiago, M. K. (2021, November). Numerical Simulations of Pulsing Soft Corals and the Photosynthesis of their Symbiotic Algae. 74th Annual Meeting of the APS Division of Fluid Dynamics. Phoenix Arizona.
- Miller, L. (2021). Flow through the hairy appendages of small animals: The leaky rake to solid plate transition.. Arizona State University Math Bio Seminar.
- Miller, L. (2021). Flow through the hairy appendages of small animals: The leaky rake to solid plate transition. Cornell Fluids Seminar.
- Miller, L. (2021). Multiscale flow between the branches and polyps of gorgonians. The 2021 Annual Meeting of the American Physical Society Division of Fluid Dynamics. Phoenix, AZ: American Physical Society.
- Miller, L. (2021). Slow and fast airflow past saguaro and other cacti. The 2021 Annual Meeting of the Society of Mathematical Biology. online: Society of Mathematical Biology.
- Miller, L. (2021). The fluid dynamics of Cassiopea.. The 2021 International Cassiopea Workshop. Online.
- Miller, L. (2021). Using computational fluid dynamics to understand jellyfish swimming and feeding. Cold Place Seminar Series.
- Miller, L. (2021). Using computational fluid dynamics to understand jellyfish swimming and feeding. Interdisciplinary online seminar series on Biolocomotion.
- Miller, L. (2021). Using computational fluid dynamics to understand muscle driven movement by soft tissues and bodies: Case studies in tubular hearts and jellyfish. BME SeminarUniversity of Arizona, Department of Biomedical Engineering.
- Miller, L. (2020). Flexible clap and peel in the smallest insects. American Physical Society Division of Fluid Dynamics. online: American Physical Society.
Others
- Miller, L. (2025, January). Horses in motion: A history of the biomechanics of movement. Arizona Senior Academy.